A Reaction Mechanism Can Be Derived From The
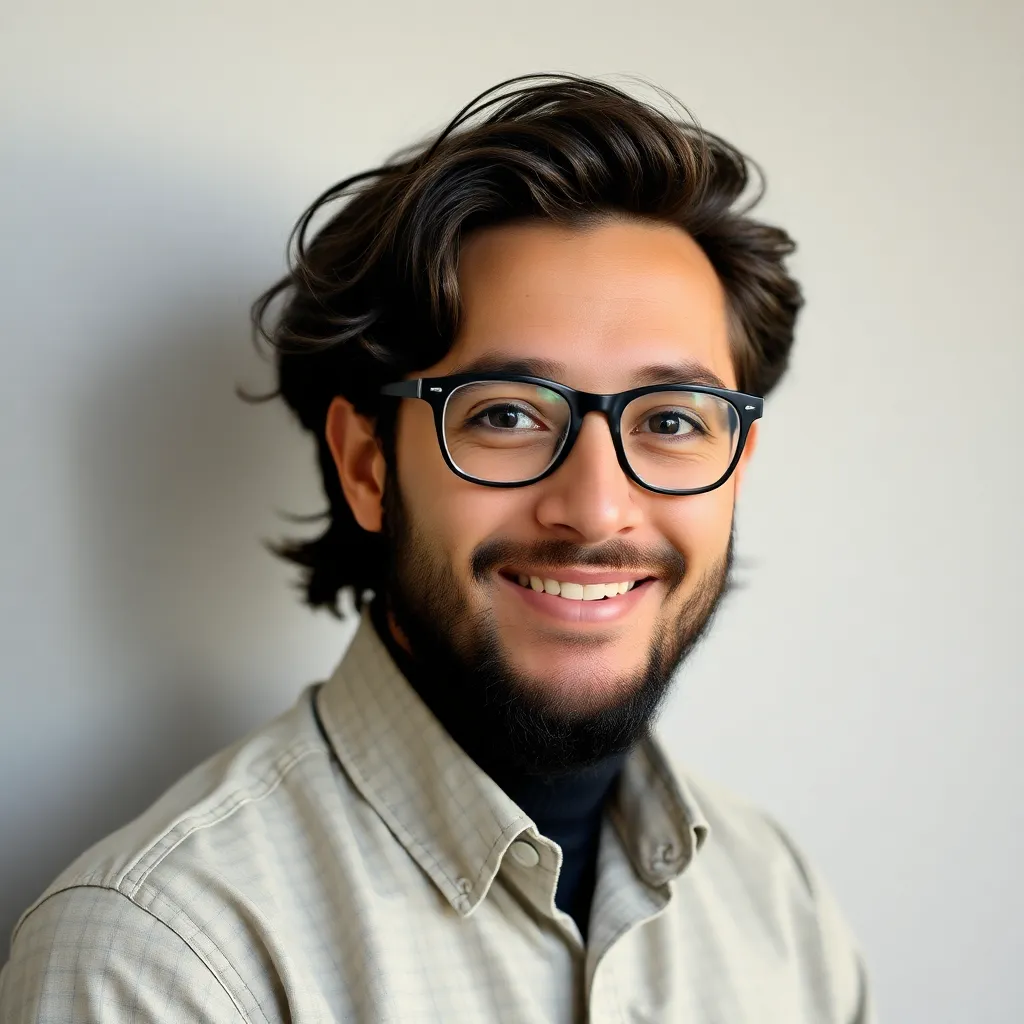
Muz Play
May 12, 2025 · 6 min read
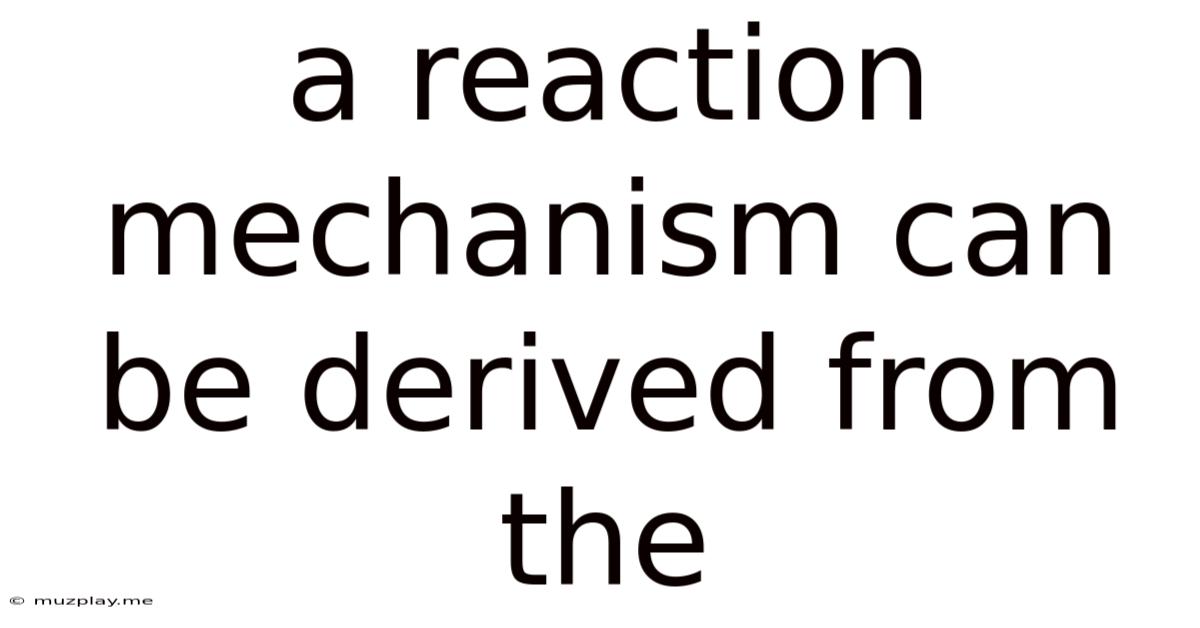
Table of Contents
A Reaction Mechanism Can Be Derived From: Unveiling the Secrets of Chemical Transformations
Understanding how chemical reactions occur is fundamental to chemistry. While we can observe the overall transformation of reactants into products, the detailed step-by-step process, known as the reaction mechanism, often remains hidden. Deriving a reaction mechanism is a crucial aspect of chemical research, allowing us to predict reaction outcomes, design new reactions, and understand the factors influencing reaction rates. But how do we unravel this intricate dance of molecules? The answer lies in a combination of experimental observations and theoretical considerations. This article delves into the various avenues through which we can deduce a reaction mechanism, highlighting the importance of each piece of evidence.
1. Kinetic Studies: The Pace of the Reaction
Kinetic studies provide invaluable insights into reaction mechanisms. By carefully measuring the rate of a reaction under various conditions, we can determine the rate law, an equation that expresses the rate of reaction as a function of reactant concentrations. The rate law's form offers critical clues about the mechanism.
1.1 Order of Reaction: Unveiling the Elementary Steps
The order of reaction with respect to each reactant, determined from the rate law, reveals how many molecules of each reactant participate in the rate-determining step (RDS), the slowest step in the mechanism. For example, a first-order reaction with respect to a particular reactant suggests that only one molecule of that reactant is involved in the RDS. A second-order reaction suggests two molecules of the same reactant or one molecule each of two different reactants.
1.2 Rate Constants and Activation Energy: A Glimpse into the Transition State
The rate constant (k), a proportionality constant in the rate law, reflects the reaction's speed. Its temperature dependence, governed by the Arrhenius equation, yields the activation energy (Ea). A high activation energy indicates a high-energy transition state, suggesting a complex mechanism. The activation energy provides information about the energy barrier that reactants must overcome to form products.
1.3 Isotope Effects: Tracing Atomic Journeys
Isotope effects provide further insights. By replacing an atom in a reactant with its heavier isotope (e.g., replacing hydrogen with deuterium), we can observe changes in the reaction rate. A significant change indicates that the bond involving the substituted atom is broken or formed in the RDS.
2. Spectroscopic Techniques: Observing the Intermediates
Reaction mechanisms often involve intermediates, short-lived species that are neither reactants nor products. Spectroscopic techniques, such as infrared (IR), nuclear magnetic resonance (NMR), and ultraviolet-visible (UV-Vis) spectroscopy, can detect these fleeting species, providing direct evidence for their existence and structure. These techniques enable us to identify the functional groups present in the intermediates and track their formation and disappearance during the reaction.
3. Product Analysis: Deciphering the Outcome
The products formed during a reaction provide crucial information about the reaction pathway. Detailed analysis of the products, including their structure and stereochemistry, can confirm or refute proposed mechanisms. Unexpected products may suggest unforeseen steps in the mechanism. Stereochemical analysis is particularly valuable for revealing the spatial arrangement of atoms during the reaction, shedding light on the stereospecificity or stereoselectivity of the reaction.
4. Computational Chemistry: The Theoretical Approach
Computational chemistry has revolutionized our ability to study reaction mechanisms. Using sophisticated software and powerful computers, chemists can model reactions in silico, simulating the transition states, intermediates, and activation energies. This allows for the investigation of reaction pathways that are difficult or impossible to study experimentally, providing valuable insights into reaction mechanisms. Computational methods are particularly useful for reactions involving complex molecules or multiple steps.
5. Stereochemistry: Unveiling the Spatial Arrangement
Stereochemistry plays a crucial role in elucidating reaction mechanisms. The spatial arrangement of atoms in the reactants and products is highly informative. For instance, a reaction that leads to a specific stereoisomer provides evidence for a stereospecific mechanism, indicating a concerted process where bond breaking and formation occur simultaneously. In contrast, a reaction producing a mixture of stereoisomers suggests a stepwise mechanism involving intermediates with free rotation.
6. Hammett Plots and Linear Free Energy Relationships (LFERs): Understanding Substituent Effects
Hammett plots and LFERs provide valuable information about the electronic effects of substituents on reaction rates. By systematically changing the substituents on a reactant and measuring the corresponding rate constants, we can determine the sensitivity of the reaction to electronic changes. This helps to identify the nature of the transition state and the role of charge development during the reaction.
7. Solvent Effects: The Influence of the Medium
The solvent in which a reaction is carried out can significantly affect the reaction rate and mechanism. Solvent effects can be used to distinguish between different mechanisms. For example, reactions involving charged intermediates are often significantly accelerated in polar solvents, whereas reactions involving neutral intermediates may be less sensitive to solvent polarity.
8. Pressure Effects: Exploring the Volume Changes
Changes in pressure can also affect reaction rates. By measuring the reaction rate at different pressures, we can determine the activation volume, which reflects the volume changes associated with the formation of the transition state. A negative activation volume suggests that the transition state is more compact than the reactants, while a positive activation volume indicates the opposite.
Putting It All Together: Constructing a Plausible Mechanism
Deriving a reaction mechanism is an iterative process. It's rarely a case of finding a single piece of definitive evidence but rather a careful compilation of various data points. A plausible mechanism should be consistent with all experimental observations, including the rate law, product distribution, spectroscopic data, stereochemical outcomes, and theoretical calculations. Moreover, a sound mechanism should be logically consistent and provide a chemically reasonable explanation for the observed phenomena. It’s crucial to remember that a mechanism is a model, a representation of reality, and it’s always subject to refinement as new evidence emerges.
Examples of Reaction Mechanisms Derived from Experimental Data
Let's consider a few examples to illustrate how these methods are used in practice:
-
SN1 and SN2 reactions: The different kinetics (first-order for SN1, second-order for SN2) and stereochemical outcomes (racemization for SN1, inversion of configuration for SN2) clearly distinguish these substitution mechanisms.
-
E1 and E2 eliminations: Similar to substitution reactions, elimination reactions also exhibit distinct kinetic behavior and stereochemical preferences that allow for the differentiation of E1 (first-order) and E2 (second-order) pathways. Isotope effects can further corroborate these distinctions.
-
Free radical reactions: The characteristic chain propagation steps, involving radical intermediates, and the influence of inhibitors and initiators strongly suggest radical mechanisms. The use of Electron Paramagnetic Resonance (EPR) spectroscopy can provide direct detection of these radical intermediates.
-
Pericyclic reactions: The concerted nature of pericyclic reactions (like Diels-Alder reactions) can often be deduced from stereochemical considerations and the absence of any detectable intermediates. Woodward-Hoffmann rules provide a theoretical framework for understanding the stereoselectivity of such reactions.
Conclusion: The Ongoing Pursuit of Understanding
Deriving a reaction mechanism is a multifaceted endeavor requiring a combination of experimental techniques and theoretical approaches. Each piece of evidence—from kinetics and spectroscopy to stereochemistry and computational chemistry—plays a vital role in constructing a plausible and accurate representation of the intricate dance of molecules that leads to the transformation of reactants into products. This pursuit of understanding is crucial not only for expanding our fundamental knowledge of chemistry but also for driving innovations in areas such as drug discovery, materials science, and catalysis. The continual refinement of experimental techniques and theoretical models promises further breakthroughs in our understanding of reaction mechanisms, unveiling even deeper secrets of chemical transformations.
Latest Posts
Latest Posts
-
Composition Of Functions Domain And Range
May 12, 2025
-
Project A Vector Onto A Plane
May 12, 2025
-
Why Is Water Referred To As The Universal Solvent
May 12, 2025
-
Parts And Names Of A Microscope
May 12, 2025
-
Examples Of Implementation In Nursing Process
May 12, 2025
Related Post
Thank you for visiting our website which covers about A Reaction Mechanism Can Be Derived From The . We hope the information provided has been useful to you. Feel free to contact us if you have any questions or need further assistance. See you next time and don't miss to bookmark.