Active Sites On The Actin Become Available For Binding After
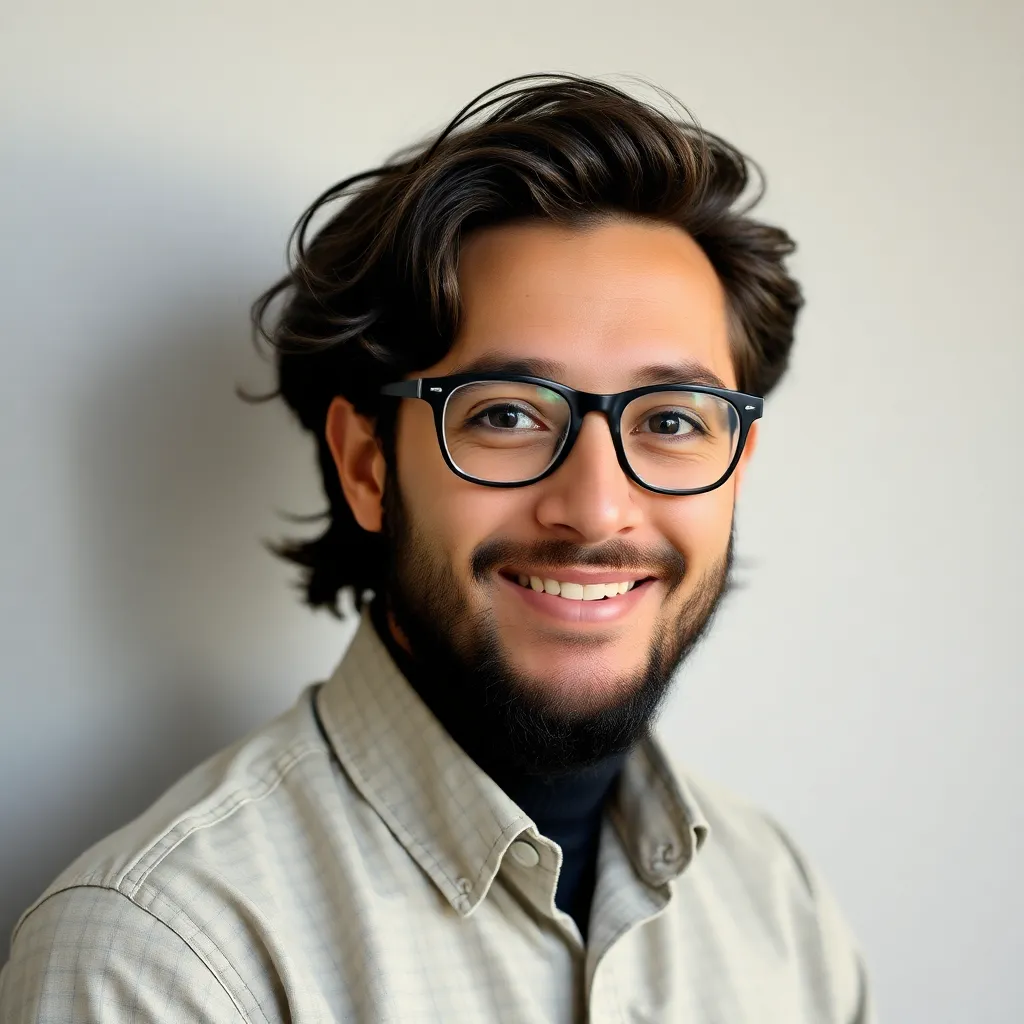
Muz Play
Apr 01, 2025 · 6 min read
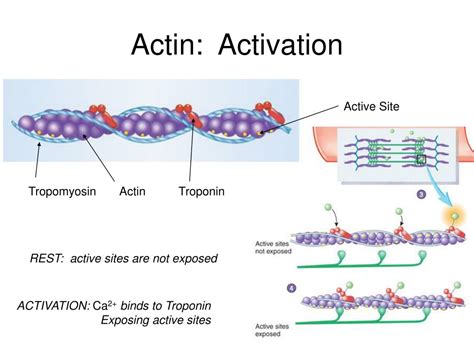
Table of Contents
Active Sites on the Actin Become Available for Binding After: Conformational Changes and Regulatory Mechanisms
Actin, a ubiquitous and highly conserved protein, plays a crucial role in a multitude of cellular processes, including cell motility, cytokinesis, and intracellular transport. Its ability to polymerize into filaments, known as F-actin, is tightly regulated and depends on the availability of active sites on the actin monomer (G-actin) for binding to other monomers. Understanding how these active sites become available is key to comprehending the dynamics of actin polymerization and its regulatory mechanisms. This article delves into the conformational changes and regulatory mechanisms that govern the accessibility of actin's active sites, impacting the overall dynamics of the actin cytoskeleton.
The Actin Monomer: Structure and Active Sites
The globular actin monomer (G-actin) possesses a cleft between two domains, the large and small domains. This cleft houses the active site, crucial for ATP binding and hydrolysis, and the interaction with other actin monomers during polymerization. The precise arrangement of amino acid residues within this cleft dictates the affinity for ATP and the ability to interact with neighboring actin molecules. This interaction is crucial for the formation of F-actin filaments.
ATP Binding and Hydrolysis: A Key Regulatory Step
G-actin binds ATP, which is then hydrolyzed to ADP during the polymerization process. This hydrolysis is not a prerequisite for polymerization per se, but it significantly influences the dynamics and stability of the filament. The ATP-bound form of G-actin generally exhibits a higher affinity for other monomers, promoting faster polymerization rates. The ADP-bound form, on the other hand, tends to be more readily released from the filament, leading to depolymerization. This ATP-hydrolysis cycle, therefore, acts as a crucial regulatory mechanism influencing the overall length and stability of actin filaments.
Conformational Changes Upon Nucleotide Binding and Hydrolysis
The binding and hydrolysis of ATP induce significant conformational changes within the actin monomer. These changes alter the accessibility of the active site, influencing its ability to interact with other actin monomers. Specifically, ATP binding leads to a more open conformation of the cleft, increasing its affinity for binding to other monomers. Conversely, ADP binding leads to a more closed conformation, reducing the affinity for further monomer addition. These conformational shifts are not simply on/off switches; they represent a spectrum of intermediate states, each contributing to the complex dynamics of actin polymerization.
Regulatory Proteins: Shaping Actin Dynamics
The availability of active sites on actin is not solely determined by the nucleotide state. A variety of regulatory proteins further modulate actin polymerization by influencing the conformational state of G-actin and the accessibility of its active sites.
Profilin: Facilitating Monomer Addition
Profilin is a ubiquitous actin-binding protein that plays a crucial role in enhancing the rate of actin polymerization. It binds to the ADP-G-actin complex and catalyzes the exchange of ADP for ATP, effectively converting ADP-G-actin into the high-affinity ATP-G-actin form. This exchange facilitates the addition of monomers to the growing filament ends. Additionally, profilin binds to the region of G-actin that usually interacts with the filament, preventing self-association of monomers and thereby indirectly enhancing the availability of the active site for interaction with the growing filament.
Thymosin β4: Sequestering Actin Monomers
In contrast to profilin, thymosin β4 sequesters G-actin monomers, preventing their participation in polymerization. It binds to ATP-G-actin, masking its active site and preventing its addition to the filament. By buffering the concentration of polymerizable G-actin, thymosin β4 regulates the availability of monomers for filament growth. The balance between profilin and thymosin β4 activity thus plays a crucial role in controlling the overall rate of actin polymerization.
Formins: Nucleating Filament Growth
Formins are a class of proteins that act as nucleators, initiating the formation of new actin filaments. They possess a unique mechanism for promoting filament elongation. They bind to the barbed end of the growing filament, remaining associated with it as it extends. By associating with the barbed end, formins effectively protect the active site from capping proteins, allowing for rapid and efficient filament elongation.
Arp2/3 Complex: Branching of Actin Filaments
The Arp2/3 complex is another crucial regulator of actin polymerization. This complex nucleates the formation of new filaments from the side of pre-existing filaments, generating branched structures. It does this by mimicking the conformation of two actin monomers, thus providing a template for new filament formation. The precise mechanisms by which Arp2/3 complex regulates the availability of active sites are still under investigation, but it’s clear that its activity significantly influences the architecture of the actin cytoskeleton.
Post-Translational Modifications: Fine-Tuning Actin Function
Post-translational modifications (PTMs) of actin, such as phosphorylation and acetylation, can also influence the availability of its active sites and hence regulate its function. These modifications can affect the conformation of actin monomers, altering their interaction with other proteins and influencing their incorporation into filaments. For example, phosphorylation of specific residues has been shown to modulate actin's interaction with profilin, thereby influencing the rate of polymerization.
Diseases Associated with Actin Dysfunction
The precise regulation of actin polymerization and depolymerization is crucial for normal cellular function. Disruptions in these processes, resulting from mutations in actin itself or its regulatory proteins, can lead to a variety of diseases.
Muscular Dystrophies
Mutations in actin or its associated proteins are frequently implicated in muscular dystrophies. These mutations often compromise the integrity of the actin cytoskeleton in muscle cells, leading to muscle weakness and degeneration. The precise mechanisms by which these mutations affect actin function are complex and often involve alterations in filament stability and interactions with other cytoskeletal proteins.
Neurological Disorders
Actin dysfunction is also linked to several neurological disorders. For instance, defects in actin dynamics can affect neuronal morphology, synapse formation, and axonal transport, all of which are essential for proper brain function. These defects can contribute to neurological disorders such as Alzheimer’s disease and Parkinson’s disease.
Cancer
Actin plays a critical role in cell motility and invasion, processes that are hallmarks of cancer metastasis. Changes in actin dynamics, often driven by mutations in actin-regulating proteins, contribute to the increased motility and invasiveness of cancer cells. Understanding the precise mechanisms involved could lead to the development of new therapeutic strategies targeting cancer metastasis.
Concluding Remarks
The availability of active sites on the actin monomer is a tightly controlled process involving a complex interplay of conformational changes, nucleotide binding and hydrolysis, and interactions with numerous regulatory proteins. These processes work in concert to regulate the dynamics of actin polymerization, thereby influencing a vast array of cellular processes. Disruptions in this finely tuned regulatory system can have significant consequences, contributing to a wide range of diseases. Further research into the intricate mechanisms governing actin dynamics is crucial for advancing our understanding of cellular processes and developing effective therapeutic strategies for diseases associated with actin dysfunction. The field continues to evolve, with ongoing investigation into the precise conformational changes, the roles of less-studied regulatory proteins, and the impact of various PTMs on actin function. A deeper understanding of this complex interplay is essential for unlocking new avenues in biomedical research and therapeutic development.
Latest Posts
Latest Posts
-
How Do Decomposers Get Their Energy
Apr 02, 2025
-
Stabilizing And Disruptive Selection Worksheet Answers
Apr 02, 2025
-
Evaluate The Following Integral In Cylindrical Coordinates
Apr 02, 2025
-
What Forms When Two Or More Atoms Bond Together
Apr 02, 2025
-
Which Organelles Are Found In Only Plant Cells
Apr 02, 2025
Related Post
Thank you for visiting our website which covers about Active Sites On The Actin Become Available For Binding After . We hope the information provided has been useful to you. Feel free to contact us if you have any questions or need further assistance. See you next time and don't miss to bookmark.