After Drawing A Lewis Structure One Should
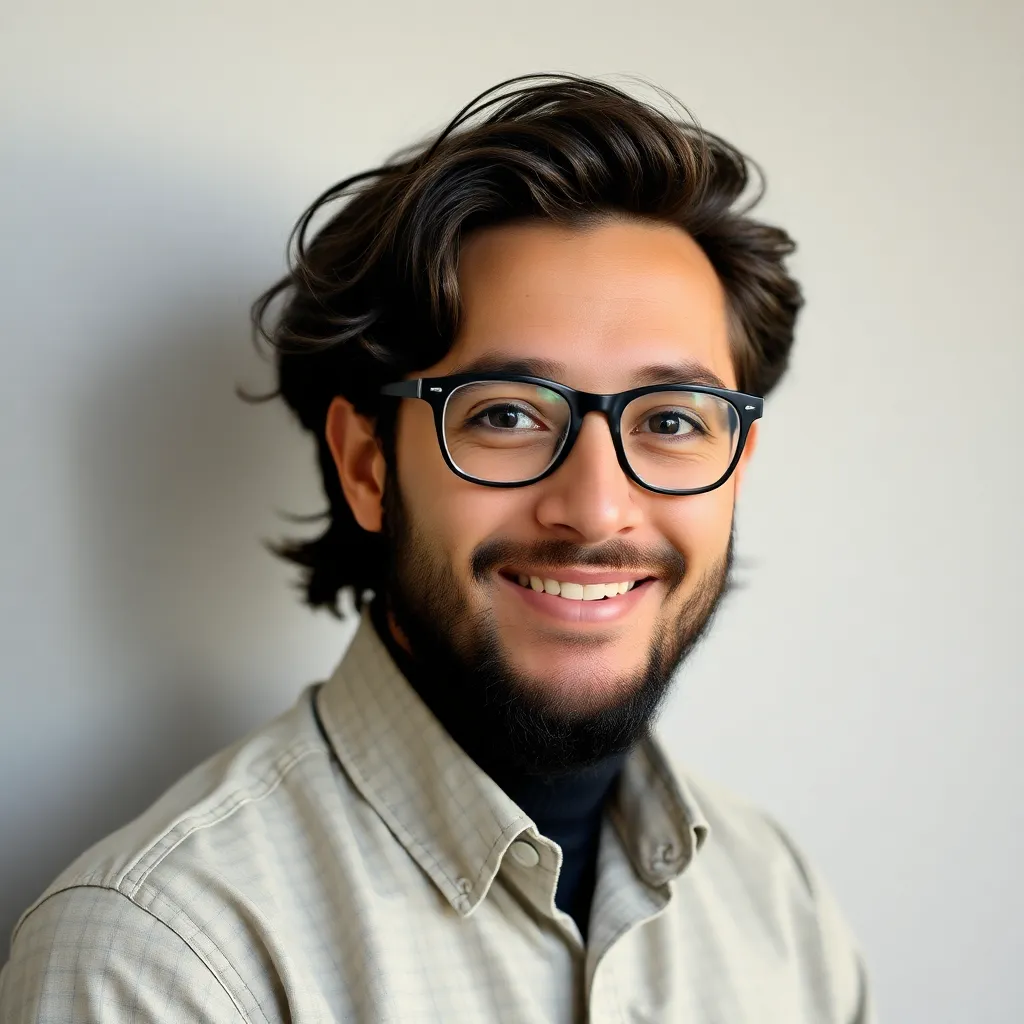
Muz Play
Apr 17, 2025 · 7 min read

Table of Contents
After Drawing a Lewis Structure: What to Do Next
Drawing a Lewis structure is a crucial first step in understanding the bonding and properties of a molecule. However, the Lewis structure itself is only a starting point. Once you've successfully drawn the Lewis structure, several important analyses can be performed to gain a deeper understanding of the molecule's characteristics. This process isn't just about following steps; it's about developing a conceptual understanding of molecular behavior. This article will guide you through those crucial next steps, from determining molecular geometry to predicting polarity and reactivity.
1. Determine the Molecular Geometry (Shape)
The Lewis structure provides the connectivity of atoms, showing which atoms are bonded to each other. However, it doesn't explicitly show the three-dimensional arrangement of atoms in space. This three-dimensional arrangement, called the molecular geometry or shape, significantly impacts the molecule's properties. We use theories like VSEPR (Valence Shell Electron Pair Repulsion) theory to predict the geometry.
Understanding VSEPR Theory
VSEPR theory postulates that electron pairs, both bonding and lone pairs, repel each other and arrange themselves to minimize this repulsion. This arrangement determines the molecular geometry. The central atom is the key focus here. Count the number of electron domains around the central atom. An electron domain can be a single bond, a double bond, a triple bond, or a lone pair of electrons.
- 2 electron domains: Linear geometry (e.g., BeCl<sub>2</sub>)
- 3 electron domains: Trigonal planar geometry (e.g., BF<sub>3</sub>)
- 4 electron domains: Tetrahedral geometry (e.g., CH<sub>4</sub>) or Trigonal pyramidal (e.g., NH<sub>3</sub>) if one domain is a lone pair. Bent geometry (e.g., H<sub>2</sub>O) if two domains are lone pairs.
- 5 electron domains: Trigonal bipyramidal geometry (e.g., PCl<sub>5</sub>)
- 6 electron domains: Octahedral geometry (e.g., SF<sub>6</sub>)
Important Note: Lone pairs occupy more space than bonding pairs, influencing the bond angles and overall shape. For example, the bond angle in water (H<sub>2</sub>O) is less than 109.5° (the ideal tetrahedral angle) due to the repulsion exerted by the two lone pairs on the oxygen atom.
Visualizing Molecular Geometry
Once you've determined the number of electron domains and lone pairs, you can use various resources (textbooks, online tools) to visualize the predicted molecular geometry. Understanding the 3D structure is essential for predicting properties like polarity and reactivity.
2. Determine the Hybridization of the Central Atom
Hybridization is a crucial concept that explains the bonding in molecules. It describes the mixing of atomic orbitals to form new hybrid orbitals that are involved in bonding. The number and type of hybrid orbitals depend on the number of electron domains around the central atom.
- 2 electron domains: sp hybridization (linear)
- 3 electron domains: sp<sup>2</sup> hybridization (trigonal planar)
- 4 electron domains: sp<sup>3</sup> hybridization (tetrahedral)
- 5 electron domains: sp<sup>3</sup>d hybridization (trigonal bipyramidal)
- 6 electron domains: sp<sup>3</sup>d<sup>2</sup> hybridization (octahedral)
Knowing the hybridization helps understand the molecule's bonding characteristics and shape, providing additional detail beyond the basic VSEPR geometry prediction. It's a powerful tool for explaining the observed bond angles and overall molecular structure.
3. Determine the Polarity of the Molecule
Molecular polarity arises from the unequal distribution of electron density within the molecule. This unequal distribution is caused by differences in electronegativity between atoms. Electronegativity is a measure of an atom's ability to attract electrons in a bond.
Electronegativity Differences and Bond Polarity
A difference in electronegativity between two bonded atoms creates a polar bond, with a partial positive charge (δ+) on the less electronegative atom and a partial negative charge (δ-) on the more electronegative atom.
Molecular Dipole Moment
Even if individual bonds are polar, the entire molecule may be nonpolar if the polar bonds cancel each other out due to symmetry. The molecular dipole moment is a vector quantity that represents the overall polarity of the molecule. If the dipole moments of individual bonds cancel, the molecule is nonpolar (e.g., CO<sub>2</sub>). If they don't cancel, the molecule is polar (e.g., H<sub>2</sub>O).
Predicting Molecular Polarity
To predict molecular polarity:
- Determine the polarity of individual bonds: Check the electronegativity difference between bonded atoms.
- Consider the molecular geometry: Determine if the polar bonds cancel each other out based on the symmetry of the molecule.
- Determine the molecular dipole moment: A non-zero dipole moment indicates a polar molecule.
Understanding molecular polarity is essential because it influences many physical and chemical properties, such as boiling point, solubility, and reactivity.
4. Determine Formal Charges
Formal charges are a bookkeeping device that helps determine the most stable Lewis structure for a molecule or ion. It is the charge assigned to an atom in a molecule, assuming that electrons in all chemical bonds are shared equally between atoms.
Calculating Formal Charge:
Formal charge = (Valence electrons) - (Non-bonding electrons) - ½(Bonding electrons)
A formal charge of zero is ideal, indicating the most stable arrangement of electrons. However, some molecules may exhibit non-zero formal charges on specific atoms. The best Lewis structure is the one that minimizes the formal charges on all atoms and places negative formal charges on the more electronegative atoms.
5. Identify Resonance Structures
Some molecules can be represented by multiple Lewis structures, each differing only in the placement of electrons. These are called resonance structures. Resonance structures don't represent different molecules; instead, they represent different ways of depicting the same molecule's delocalized electron distribution. The actual molecule is a hybrid of all resonance structures. Resonance stabilization significantly impacts the molecule's stability and reactivity. The molecule's true structure is a resonance hybrid, a weighted average of the contributing resonance structures.
6. Predict the Bond Order
Bond order refers to the number of chemical bonds between a pair of atoms. A single bond has a bond order of 1, a double bond has a bond order of 2, and a triple bond has a bond order of 3. In molecules with resonance structures, the bond order is the average of the bond orders in the contributing resonance structures. Bond order is directly related to bond length and bond strength: higher bond order implies shorter and stronger bonds.
7. Analyze the Oxidation States
The oxidation state (or oxidation number) of an atom represents the hypothetical charge it would have if all bonds were completely ionic. This is a useful concept for understanding redox reactions (reduction-oxidation reactions) where electron transfer takes place. Determining the oxidation states of atoms in a molecule helps understand the electron transfer process during chemical reactions. Remember, oxidation states are hypothetical charges, not actual charges.
8. Predict Reactivity based on Lewis Structure
The Lewis structure provides valuable insight into a molecule's reactivity. Factors like the presence of lone pairs, multiple bonds, and formal charges influence a molecule's ability to participate in chemical reactions. For example, molecules with lone pairs on electronegative atoms are often good Lewis bases (electron donors), while molecules with electron-deficient atoms might act as Lewis acids (electron acceptors). The presence of multiple bonds indicates potential sites for addition reactions, and the presence of strained rings or unusual bond angles can also affect reactivity.
9. Compare to Experimental Data
The predictions made based on the Lewis structure, VSEPR theory, and other theoretical models must be compared with experimental data. Techniques like X-ray crystallography and spectroscopy provide experimental evidence about molecular structure, bond lengths, bond angles, and other properties. Comparing these experimental results with theoretical predictions helps refine our understanding of molecular structure and bonding. Discrepancies can highlight limitations in the models and point to the need for more sophisticated theoretical treatments.
Conclusion: Beyond the Structure
Drawing a Lewis structure is just the first step in a comprehensive analysis of a molecule. By applying VSEPR theory, determining hybridization, predicting polarity, calculating formal charges, and analyzing bond order and oxidation states, you gain a much deeper understanding of the molecule's properties and reactivity. Remember to always compare your predictions with experimental data to validate your understanding and refine your theoretical models. This holistic approach is crucial for mastering chemical bonding and gaining a strong foundation in chemistry.
Latest Posts
Latest Posts
-
How Many Protons And Neutrons Does Boron Have
Apr 19, 2025
-
In The Process Of Science Which Of These Is Tested
Apr 19, 2025
-
Examples Of Arguments In Everyday Life
Apr 19, 2025
-
How Many Parents Are Involved In Asexual Reproduction
Apr 19, 2025
-
Which Of The Following Does The Enzyme Primase Synthesize
Apr 19, 2025
Related Post
Thank you for visiting our website which covers about After Drawing A Lewis Structure One Should . We hope the information provided has been useful to you. Feel free to contact us if you have any questions or need further assistance. See you next time and don't miss to bookmark.