Along A Neuron The Correct Pathway For Impulse Conduction Is
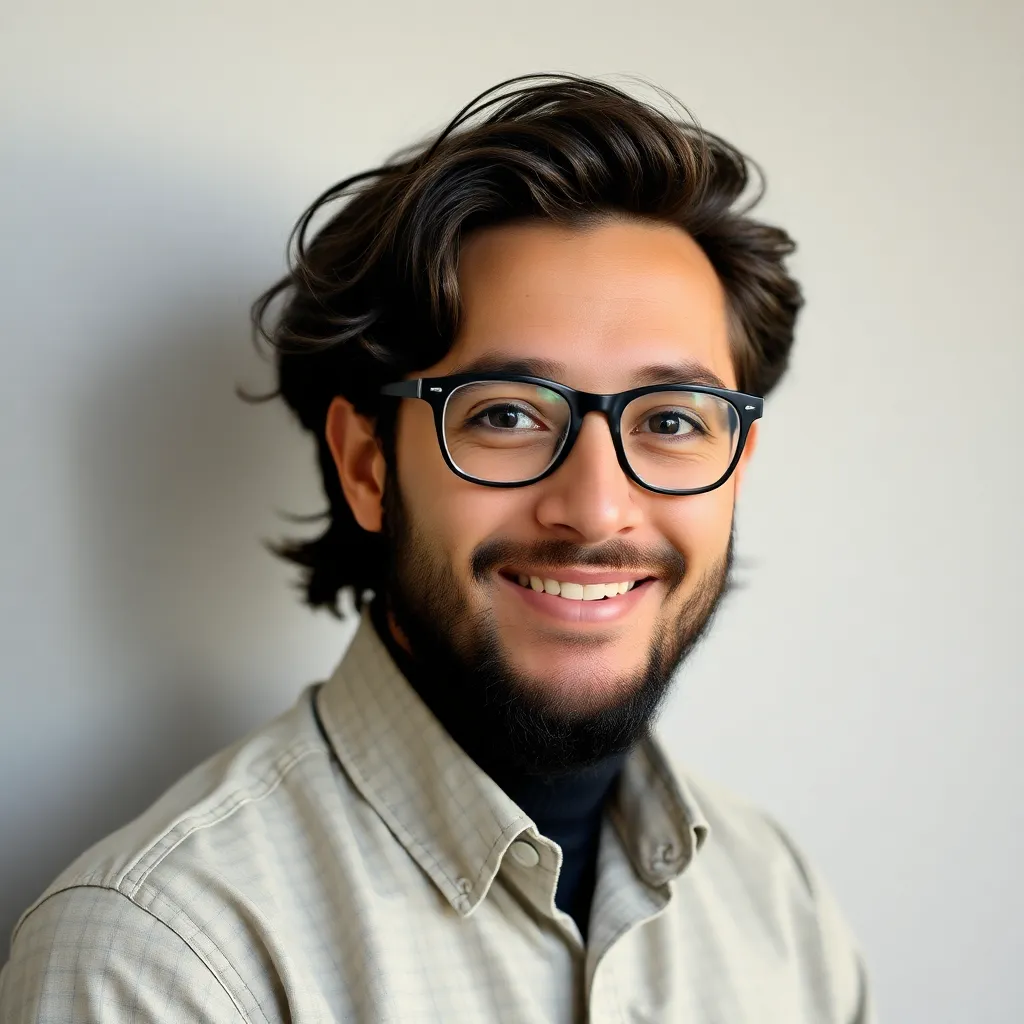
Muz Play
May 11, 2025 · 7 min read

Table of Contents
Along a Neuron: The Correct Pathway for Impulse Conduction
The human nervous system, a marvel of biological engineering, relies on the precise transmission of electrical signals, or nerve impulses, to control virtually every aspect of our physiology and behavior. This transmission hinges on the intricate structure and function of neurons, the fundamental units of the nervous system. Understanding the correct pathway for impulse conduction along a neuron is crucial to grasping how our brains, spinal cords, and peripheral nerves orchestrate our actions, sensations, and thoughts.
The Neuron: A Cellular Messenger
Before delving into the pathway of impulse conduction, let's establish a foundational understanding of the neuron's structure. A neuron, unlike many other cells, is highly specialized for the rapid and efficient transmission of information. Its key components include:
1. Dendrites: The Receiving Antennae
Dendrites are branching, tree-like structures extending from the neuron's cell body (soma). They act as the neuron's primary receivers, collecting incoming signals from other neurons. These signals are primarily chemical in nature, transmitted across tiny gaps called synapses. The dendrites' vast surface area maximizes the number of synaptic connections a single neuron can make, amplifying its capacity for information processing. The intricate branching pattern ensures that even subtle signals can be effectively detected and integrated.
2. Soma (Cell Body): The Integration Center
The soma, or cell body, contains the neuron's nucleus and other essential organelles. It acts as the neuron's metabolic center, responsible for maintaining cellular functions. More importantly, it integrates the incoming signals received by the dendrites. The soma sums up these signals – some excitatory (promoting impulse generation) and some inhibitory (suppressing impulse generation) – to determine whether the neuron will fire an impulse. This integration is a crucial step in information processing, allowing the neuron to respond selectively to specific patterns of input.
3. Axon: The Transmission Cable
The axon is a long, slender projection extending from the soma. It is the neuron's primary transmitter, responsible for conducting the nerve impulse over long distances to other neurons, muscles, or glands. The axon's structure is critical for efficient impulse conduction. Many axons are myelinated, meaning they are coated with a fatty substance called myelin, formed by specialized glial cells (oligodendrocytes in the central nervous system and Schwann cells in the peripheral nervous system). Myelin acts as an insulator, increasing the speed of impulse conduction significantly. The gaps between myelin sheaths are known as Nodes of Ranvier, which play a crucial role in saltatory conduction, a mechanism we'll explore later.
4. Axon Terminals (Synaptic Boutons): The Signal Transmitters
At the end of the axon, the axon branches into numerous fine terminals called axon terminals or synaptic boutons. These terminals form synapses with other neurons or effector cells (muscle or gland cells). At the synapse, the nerve impulse is converted into a chemical signal, typically neurotransmitters, which are released into the synaptic cleft (the gap between the axon terminal and the target cell). These neurotransmitters bind to receptors on the target cell, initiating a response in the target cell, thus continuing the propagation of the signal.
The Pathway of Impulse Conduction: A Step-by-Step Guide
The correct pathway of impulse conduction is a fascinating interplay of electrical and chemical events. It can be broken down into these stages:
1. Reception of Signals at Dendrites: The Initial Spark
The process begins with the reception of signals at the dendrites. These signals are typically chemical, arising from neurotransmitters released by other neurons at synapses. Neurotransmitter binding to receptors on the dendrites opens ion channels, altering the membrane potential of the dendrite. If the net effect of these changes is to depolarize the membrane (make it less negative), the signal is excitatory. Conversely, if the membrane becomes more negative (hyperpolarization), the signal is inhibitory.
2. Signal Integration at the Soma: Summing Up the Input
The depolarizations and hyperpolarizations resulting from synaptic input are summed up at the soma. This process, known as summation, is crucial. If the sum of excitatory postsynaptic potentials (EPSPs) exceeds a certain threshold, the neuron will fire an action potential. If the sum of inhibitory postsynaptic potentials (IPSPs) dominates, or if the total excitation does not reach the threshold, the neuron will not fire. This summation mechanism allows the neuron to act as a sophisticated decision-making unit, responding only to significant or specific patterns of input.
3. Action Potential Generation at the Axon Hillock: The All-or-Nothing Response
If the sum of EPSPs at the axon hillock (the region where the axon originates from the soma) reaches the threshold potential, an action potential is generated. This is an all-or-nothing event; either the action potential fires at full strength or it does not fire at all. The action potential is a rapid, self-propagating depolarization of the axon membrane. This depolarization is caused by the opening of voltage-gated sodium channels, allowing a rapid influx of sodium ions into the axon. This influx causes a significant change in the membrane potential, leading to the characteristic spike of an action potential.
4. Action Potential Propagation along the Axon: Saltatory Conduction and Myelin's Role
Once initiated, the action potential propagates along the axon. In myelinated axons, this propagation is significantly faster due to saltatory conduction. In this process, the action potential "jumps" from one Node of Ranvier to the next, skipping over the myelinated segments. This "jumping" significantly accelerates the transmission speed compared to unmyelinated axons where the action potential propagates continuously along the axon membrane. The myelin sheath ensures that the depolarization is highly concentrated at the Nodes of Ranvier, thus enhancing the efficiency of the signal propagation.
5. Neurotransmitter Release at the Axon Terminals: Chemical Signaling Across the Synapse
When the action potential reaches the axon terminals, it triggers the release of neurotransmitters into the synaptic cleft. This release is initiated by the opening of voltage-gated calcium channels, allowing calcium ions to enter the axon terminal. The influx of calcium ions triggers the fusion of synaptic vesicles (containing neurotransmitters) with the presynaptic membrane, resulting in the release of neurotransmitters into the synaptic cleft.
6. Signal Reception by the Postsynaptic Neuron: The Cycle Continues
The neurotransmitters diffuse across the synaptic cleft and bind to receptors on the postsynaptic neuron (or effector cell). This binding initiates a new cycle of depolarization or hyperpolarization, depending on the type of neurotransmitter and receptor involved. The process repeats itself, transmitting the signal along the neuronal pathway.
Factors Affecting Impulse Conduction Speed
Several factors influence the speed of impulse conduction:
-
Axon Diameter: Larger diameter axons offer less resistance to ion flow, leading to faster conduction speeds.
-
Myelination: Myelinated axons conduct impulses much faster than unmyelinated axons due to saltatory conduction.
-
Temperature: Higher temperatures generally lead to faster conduction speeds, as ion channels open and close more rapidly.
-
Presence of certain drugs and toxins: Some substances can interfere with ion channel function, either slowing or blocking impulse conduction.
Clinical Significance and Neurological Disorders
Disruptions to the normal pathway of impulse conduction can lead to a wide range of neurological disorders. Examples include:
-
Multiple Sclerosis (MS): An autoimmune disease that damages the myelin sheath, leading to slower and less efficient impulse conduction.
-
Guillain-Barré syndrome: An autoimmune disease affecting the peripheral nervous system, causing weakness and paralysis due to impaired impulse conduction.
-
Stroke: Disruption of blood flow to the brain can lead to neuronal death and impaired impulse conduction, resulting in neurological deficits.
-
Neuropathies: Damage to nerves caused by various factors (diabetes, alcohol abuse, etc.) can result in impaired impulse conduction and associated sensory or motor problems.
Conclusion: The Exquisite Precision of Neuronal Signaling
The pathway of impulse conduction along a neuron is a remarkably precise and efficient process. It depends on the intricate interplay of electrical and chemical events, involving the coordinated actions of numerous ion channels, neurotransmitters, and specialized glial cells. A deep understanding of this pathway is fundamental to comprehending the complexities of the nervous system and the diverse neurological functions it supports. Furthermore, recognizing the factors that can affect impulse conduction is crucial in diagnosing and treating a wide array of neurological disorders. The remarkable efficiency and precision of this cellular communication underpins our ability to perceive, act, and think—a testament to the power of biological design.
Latest Posts
Related Post
Thank you for visiting our website which covers about Along A Neuron The Correct Pathway For Impulse Conduction Is . We hope the information provided has been useful to you. Feel free to contact us if you have any questions or need further assistance. See you next time and don't miss to bookmark.