An Allosteric Enzyme That Follows The Concerted Mechanism
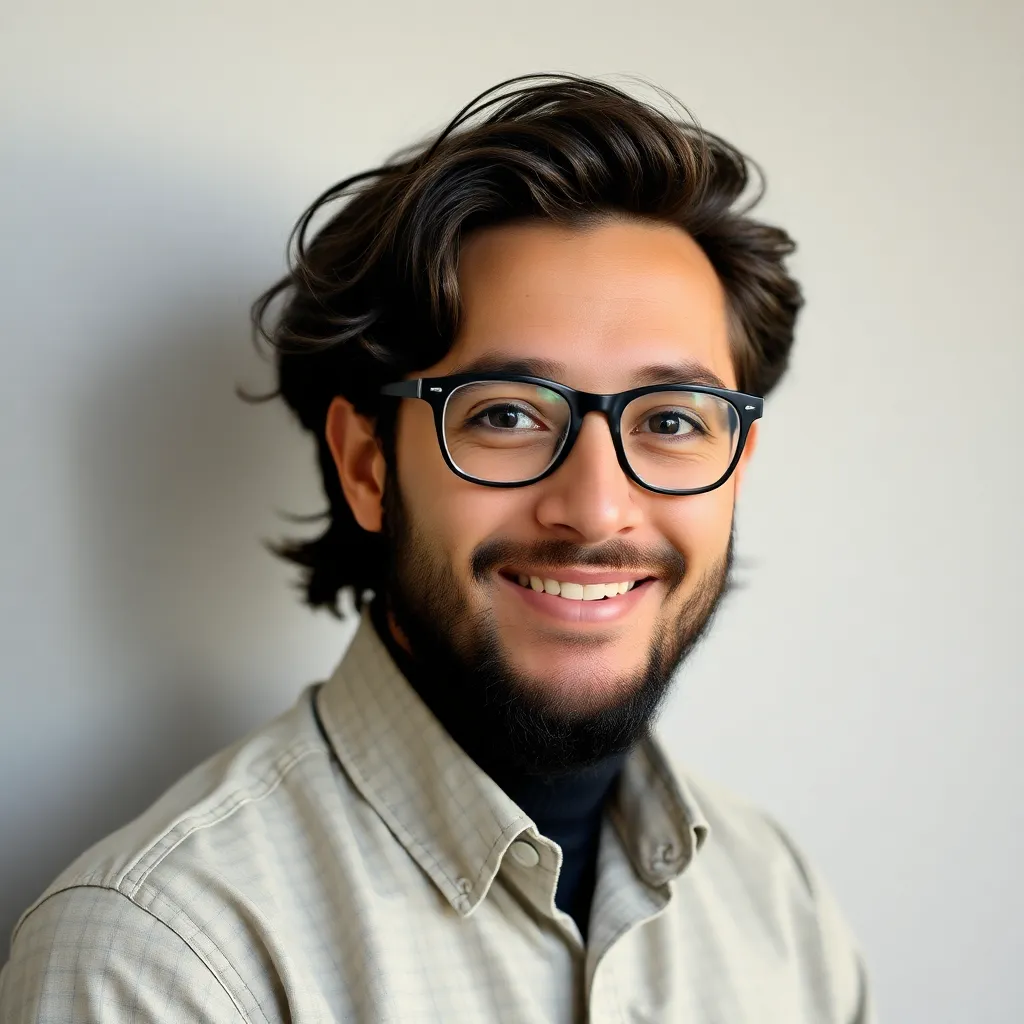
Muz Play
Apr 23, 2025 · 6 min read

Table of Contents
Allosteric Enzymes: A Deep Dive into the Concerted Mechanism
Allosteric enzymes, the dynamic regulators of metabolic pathways, are fascinating molecular machines. Their ability to switch between active and inactive conformations in response to effector molecules is crucial for maintaining cellular homeostasis. Among the models explaining this behavior, the concerted mechanism, also known as the symmetry model, stands out for its elegant simplicity and predictive power. This article delves deep into the concerted mechanism, exploring its principles, implications, and relevance in various biological processes.
Understanding Allosteric Enzymes and Their Regulation
Before diving into the concerted mechanism, let's establish a fundamental understanding of allosteric enzymes. These enzymes possess distinct regulatory sites, separate from their active sites, where effector molecules bind. These effectors can be either activators, enhancing enzyme activity, or inhibitors, reducing it. The binding of an effector at the allosteric site induces a conformational change in the enzyme, impacting the activity of the active site(s). This is a crucial mechanism for fine-tuning metabolic flux, adapting to changing cellular conditions.
Distinguishing Features of Allosteric Enzymes
Allosteric enzymes are characterized by several key features:
- Quaternary Structure: They typically consist of multiple subunits, often arranged symmetrically. This multimeric structure allows for cooperative interactions between subunits.
- Sigmoidal Kinetics: Unlike Michaelis-Menten enzymes exhibiting hyperbolic kinetics, allosteric enzymes display sigmoidal curves when plotting reaction velocity against substrate concentration. This sigmoidicity reflects the cooperative binding of substrate molecules.
- Allosteric Regulation: Their activity is modulated by the binding of effector molecules at allosteric sites, influencing the enzyme's conformation and catalytic efficiency.
- Cooperative Substrate Binding: The binding of one substrate molecule to one subunit influences the binding affinity of subsequent substrate molecules to other subunits.
The Concerted Mechanism: A Symphony of Conformational Changes
The concerted mechanism proposes that allosteric enzymes exist in two primary conformations: the relaxed (R) state and the tense (T) state. The R state is the active conformation, exhibiting high affinity for substrate, while the T state is the inactive conformation with low substrate affinity. Crucially, the concerted model postulates that all subunits within the enzyme exist in the same conformation simultaneously. There is no intermediate state where some subunits are in the R state and others in the T state.
Equilibrium Between R and T States
The equilibrium between the R and T states is influenced by several factors:
- Substrate Concentration: Substrate binding shifts the equilibrium towards the R state, promoting enzyme activity.
- Allosteric Effectors: Activators stabilize the R state, while inhibitors stabilize the T state, thus influencing the equilibrium accordingly.
- Intrinsic Properties: The inherent equilibrium constant (L) between the R and T states, reflecting the relative stability of each conformation in the absence of substrate or effectors, is an intrinsic property of the enzyme.
The equilibrium can be represented by the following equation:
T <=> R
(where K = [R]/[T])
The Role of Cooperativity in the Concerted Mechanism
The concerted mechanism elegantly explains the cooperative binding of substrate molecules. The binding of a substrate molecule to one subunit in the T state induces a conformational shift of all subunits to the R state. This transition makes it easier for subsequent substrate molecules to bind, resulting in the sigmoidal kinetics observed in allosteric enzymes. The increased substrate affinity after initial binding is the essence of positive cooperativity.
Mathematical Description of the Concerted Model
The concerted model can be described mathematically using a modified form of the Michaelis-Menten equation incorporating the equilibrium between the R and T states and the cooperativity factor. While a detailed derivation is beyond the scope of this article, the key parameters involved are:
- L: The equilibrium constant between the T and R states.
- K<sub>R</sub>: The dissociation constant of the substrate from the R state.
- c: The cooperativity factor, reflecting the extent of the conformational change induced by substrate binding.
- [S]: The substrate concentration.
A simplified representation might look like this (note that actual equations are far more complex):
v = V<sub>max</sub> * f([S], L, K<sub>R</sub>, c)
This equation, however, only provides a general idea of the complexity involved. Specific mathematical expressions can be quite intricate depending on the number of subunits and binding sites.
Experimental Evidence Supporting the Concerted Model
While the concerted model provides a neat explanation of allosteric behavior, it's crucial to acknowledge that real-world systems often display more complexity. However, several experimental observations support the key principles of the concerted mechanism:
- Sigmoidal Kinetics: The ubiquitous observation of sigmoidal kinetics in many allosteric enzymes is consistent with the concerted model’s prediction of cooperative substrate binding.
- Allosteric Effector Effects: The impact of allosteric activators and inhibitors on the kinetics aligns with the model's prediction of their influence on the R/T equilibrium.
- X-ray Crystallography: In some cases, X-ray crystallography has revealed structural changes consistent with large-scale conformational shifts between R and T states, although obtaining both states simultaneously can be challenging.
Limitations of the Concerted Mechanism
Despite its elegance and explanatory power, the concerted model does have limitations:
- Oversimplification: The assumption that all subunits transition simultaneously might be an oversimplification of the complex dynamics within the enzyme. Some evidence suggests that sequential transitions might also occur.
- Lack of Explanation for Negative Cooperativity: While the concerted model neatly accounts for positive cooperativity, explaining negative cooperativity requires modifications or alternative models.
- Difficulty in Accounting for Complex Allosteric Behavior: Some enzymes exhibit more complex allosteric regulation that cannot be easily explained by a simple two-state model.
The Sequential Model: An Alternative Perspective
An alternative model, the sequential model, proposes that the conformational change is not concerted but rather sequential. Substrate binding to one subunit can induce a conformational change in adjacent subunits, leading to a cascade of transitions. This model can better accommodate negative cooperativity and more nuanced allosteric interactions. Often, a hybrid model incorporating aspects of both concerted and sequential mechanisms might offer the most accurate representation of a specific enzyme.
The Concerted Mechanism in Biological Processes
The concerted mechanism plays a vital role in numerous biological processes, ensuring the precise regulation of metabolic pathways:
- Glycolysis: Allosteric enzymes like phosphofructokinase (PFK) are central regulators of glycolysis, responding to energy levels and substrate availability.
- Citric Acid Cycle: Enzymes involved in the citric acid cycle are also often allosterically regulated, adapting to the metabolic demands of the cell.
- Biosynthetic Pathways: Allosteric regulation ensures the efficient production of metabolites, avoiding unnecessary energy expenditure.
- Signal Transduction: Some allosteric enzymes are involved in signal transduction pathways, amplifying or attenuating signals.
Conclusion: A Powerful but Not Perfect Model
The concerted mechanism, while not a universally applicable model for all allosteric enzymes, provides a valuable framework for understanding the cooperative binding of substrates and the allosteric regulation of enzyme activity. Its simplicity and predictive power make it a cornerstone in the study of enzyme kinetics and regulation. While more complex models, such as the sequential model or hybrid models, are needed to account for the full spectrum of allosteric behavior, the concerted mechanism remains a vital tool in deciphering the intricate world of enzyme regulation. Further research continues to refine our understanding of these crucial biological processes and the underlying molecular mechanisms that govern them. The study of allosteric enzymes remains a vibrant and productive area of biochemistry, promising continued insights into the complex regulatory networks within living cells.
Latest Posts
Latest Posts
-
Compare And Contrast Condensation And Evaporation
Apr 23, 2025
-
What Is The Formula Of The Cocl2 Hydrate
Apr 23, 2025
-
How Do You Find The Equation Of A Horizontal Line
Apr 23, 2025
-
Why Are Some Solutions Better Conductors Of Electricity
Apr 23, 2025
-
Calcium Magnesium And Strontium Belong To Which Group
Apr 23, 2025
Related Post
Thank you for visiting our website which covers about An Allosteric Enzyme That Follows The Concerted Mechanism . We hope the information provided has been useful to you. Feel free to contact us if you have any questions or need further assistance. See you next time and don't miss to bookmark.