An Unstable Nucleus Results From Too Many Or Too Few
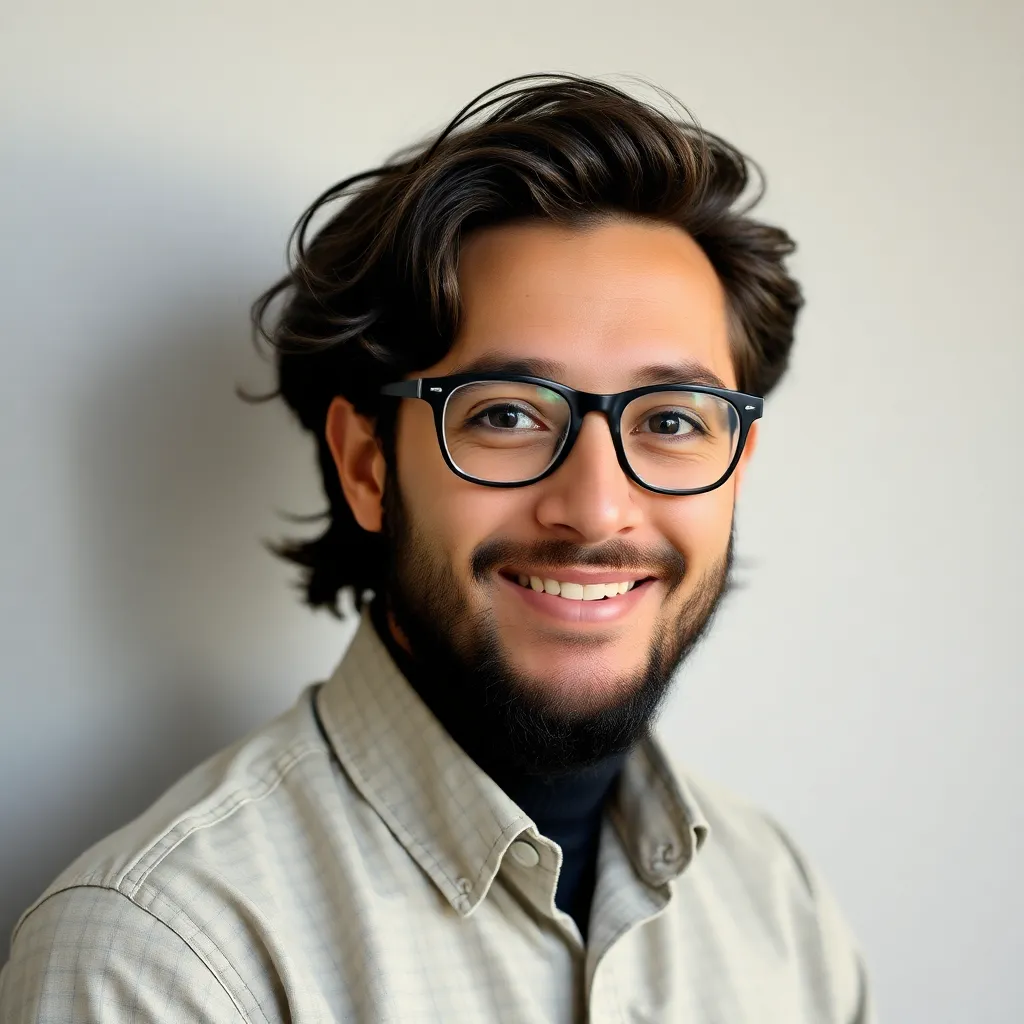
Muz Play
Mar 12, 2025 · 6 min read

Table of Contents
An Unstable Nucleus: The Consequences of Too Many or Too Few
Nuclear stability is a fascinating and crucial concept in understanding the behavior of matter at its most fundamental level. The stability of an atom's nucleus, the central core containing protons and neutrons, dictates the atom's properties and its potential to undergo radioactive decay. This article delves into the intricacies of nuclear stability, exploring why too many or too few neutrons relative to protons can lead to an unstable nucleus and the various consequences of this instability.
The Proton-Neutron Ratio: A Balancing Act
The nucleus of an atom is composed of protons and neutrons, collectively known as nucleons. Protons carry a positive charge, while neutrons are electrically neutral. The strong nuclear force, a fundamental force of nature, is responsible for binding these nucleons together, overcoming the electrostatic repulsion between the positively charged protons.
However, the strong nuclear force has a limited range. Therefore, the stability of a nucleus is intricately linked to the balance between the number of protons and neutrons. This balance is often visualized using a proton-to-neutron ratio.
For lighter elements (those with atomic numbers up to approximately 20), a stable nucleus typically has a proton-to-neutron ratio close to 1:1. This means that the number of protons and neutrons is roughly equal. For example, carbon-12 (⁶C) has six protons and six neutrons, exhibiting excellent stability.
As we move towards heavier elements, the optimal proton-to-neutron ratio shifts slightly upwards. This is because the increasing number of protons necessitates a higher proportion of neutrons to counteract the enhanced electrostatic repulsion between them and maintain nuclear stability. For larger nuclei, the number of neutrons significantly exceeds the number of protons. Lead-208 (²⁰⁸Pb), the heaviest stable isotope, has 82 protons and 126 neutrons.
The Causes of Nuclear Instability
When the proton-to-neutron ratio deviates significantly from the optimal value for a given atomic number, the nucleus becomes unstable. This instability manifests in several ways:
1. Too Many Neutrons (Neutron-Rich Nuclei):
Having too many neutrons relative to protons results in a neutron-rich nucleus. This imbalance creates an energy surplus within the nucleus, making it energetically favorable to undergo radioactive decay to achieve a more stable configuration. Common decay modes for neutron-rich nuclei include:
-
Beta-minus decay (β⁻ decay): In β⁻ decay, a neutron converts into a proton, emitting an electron (β⁻ particle) and an antineutrino. This process reduces the neutron count and increases the proton count, bringing the ratio closer to equilibrium. For example, carbon-14 (¹⁴C) undergoes β⁻ decay to become nitrogen-14 (¹⁴N).
-
Neutron emission: In some cases, the nucleus may directly eject a neutron, reducing the neutron number and achieving greater stability. This is particularly common in very neutron-rich nuclei.
2. Too Few Neutrons (Neutron-Deficient Nuclei):
Conversely, a nucleus with too few neutrons compared to its number of protons is neutron-deficient. The lack of neutrons weakens the strong nuclear force, leading to an imbalance and instability. Common decay modes for neutron-deficient nuclei include:
-
Beta-plus decay (β⁺ decay): In β⁺ decay, a proton converts into a neutron, emitting a positron (β⁺ particle, the antiparticle of an electron) and a neutrino. This process decreases the proton count and increases the neutron count, moving the proton-to-neutron ratio towards a more stable value. For instance, fluorine-18 (¹⁸F) undergoes β⁺ decay to become oxygen-18 (¹⁸O).
-
Electron capture: An alternative pathway for neutron-deficient nuclei is electron capture. In this process, the nucleus captures an inner-shell electron, which combines with a proton to form a neutron and a neutrino. This also reduces the proton count and increases the neutron count.
Consequences of Nuclear Instability: Radioactive Decay
Nuclear instability leads to radioactive decay, a spontaneous process where the unstable nucleus releases energy to achieve a more stable configuration. This release of energy can take several forms:
-
Alpha decay (α decay): In α decay, the nucleus emits an alpha particle, which consists of two protons and two neutrons (equivalent to a helium-4 nucleus). This process reduces both the proton and neutron numbers, significantly changing the identity of the atom. Alpha particles are relatively massive and have a low penetration power.
-
Gamma decay (γ decay): Gamma decay involves the emission of a gamma ray, a high-energy photon. This process doesn't change the number of protons or neutrons, but it releases excess energy from the nucleus that remains in an excited state after another decay process. Gamma rays have high penetrating power.
-
Spontaneous fission: In spontaneous fission, a heavy, unstable nucleus splits into two or more smaller nuclei, along with the release of neutrons and energy. This is a particularly energetic process, often associated with very heavy elements.
Factors Affecting Nuclear Stability Beyond the Neutron-Proton Ratio
While the proton-to-neutron ratio is a primary determinant of nuclear stability, other factors also play significant roles:
-
Pairing effect: Nuclei with even numbers of both protons and neutrons (even-even nuclei) are generally more stable than those with odd numbers of either or both. This pairing effect is due to the quantum mechanical nature of nucleons and their interactions.
-
Magic numbers: Certain specific numbers of protons or neutrons (2, 8, 20, 28, 50, 82, and 126) are associated with enhanced stability, known as "magic numbers". Nuclei with magic numbers of either protons or neutrons (or both) are exceptionally stable and tend to have longer half-lives.
-
Nuclear shell model: The nuclear shell model, analogous to the electronic shell model for atoms, provides a framework for understanding nuclear stability based on the energy levels of nucleons within the nucleus. Certain energy levels are filled, contributing to greater stability, while unfilled levels imply instability.
Applications and Implications of Nuclear Instability
The phenomenon of nuclear instability has profound implications across various fields:
-
Nuclear medicine: Radioactive isotopes are widely used in medical imaging techniques, such as PET (positron emission tomography) and SPECT (single-photon emission computed tomography), and in cancer therapy, utilizing targeted radiation to destroy cancerous cells.
-
Nuclear power: Nuclear power plants harness the energy released from controlled nuclear fission reactions to generate electricity. This relies on carefully managing the decay of unstable nuclei.
-
Radiocarbon dating: The decay of carbon-14 is utilized in radiocarbon dating, a technique used to determine the age of organic materials.
-
Geological dating: Radioactive decay of various isotopes is essential in geological dating, allowing scientists to estimate the age of rocks and minerals.
-
Nuclear forensics: Analysis of radioactive isotopes can be used in nuclear forensics investigations to identify the sources of nuclear materials and detect illicit activities.
Conclusion
The stability of an atomic nucleus hinges on a delicate balance between the number of protons and neutrons. A deviation from this optimal balance, resulting in too many or too few neutrons, leads to nuclear instability and radioactive decay. Understanding the factors governing nuclear stability is crucial not only for fundamental physics but also for numerous applications across various scientific and technological domains. The consequences of nuclear instability—from the controlled release of energy in nuclear power plants to the diagnostic use of radioactive isotopes in medicine—underscore the significance of this fundamental aspect of nuclear physics. Further research in nuclear physics continues to unravel the intricacies of nuclear stability and its implications for a wide range of applications. Continued exploration into this field remains crucial to unlock the possibilities and address the challenges associated with unstable nuclei.
Latest Posts
Latest Posts
-
How Do You Find The Center Of A Hyperbola
May 09, 2025
-
Does The Process Of Glycolysis Require An Input Of Energy
May 09, 2025
-
Difference Between Consumer And Producer Surplus
May 09, 2025
-
Dsm 5 Is The Classification System For Abnormal Behaviors That Is
May 09, 2025
-
Why Are Proteins Considered Polymers But Lipids Not
May 09, 2025
Related Post
Thank you for visiting our website which covers about An Unstable Nucleus Results From Too Many Or Too Few . We hope the information provided has been useful to you. Feel free to contact us if you have any questions or need further assistance. See you next time and don't miss to bookmark.