Basic Building Block Of The Nervous System
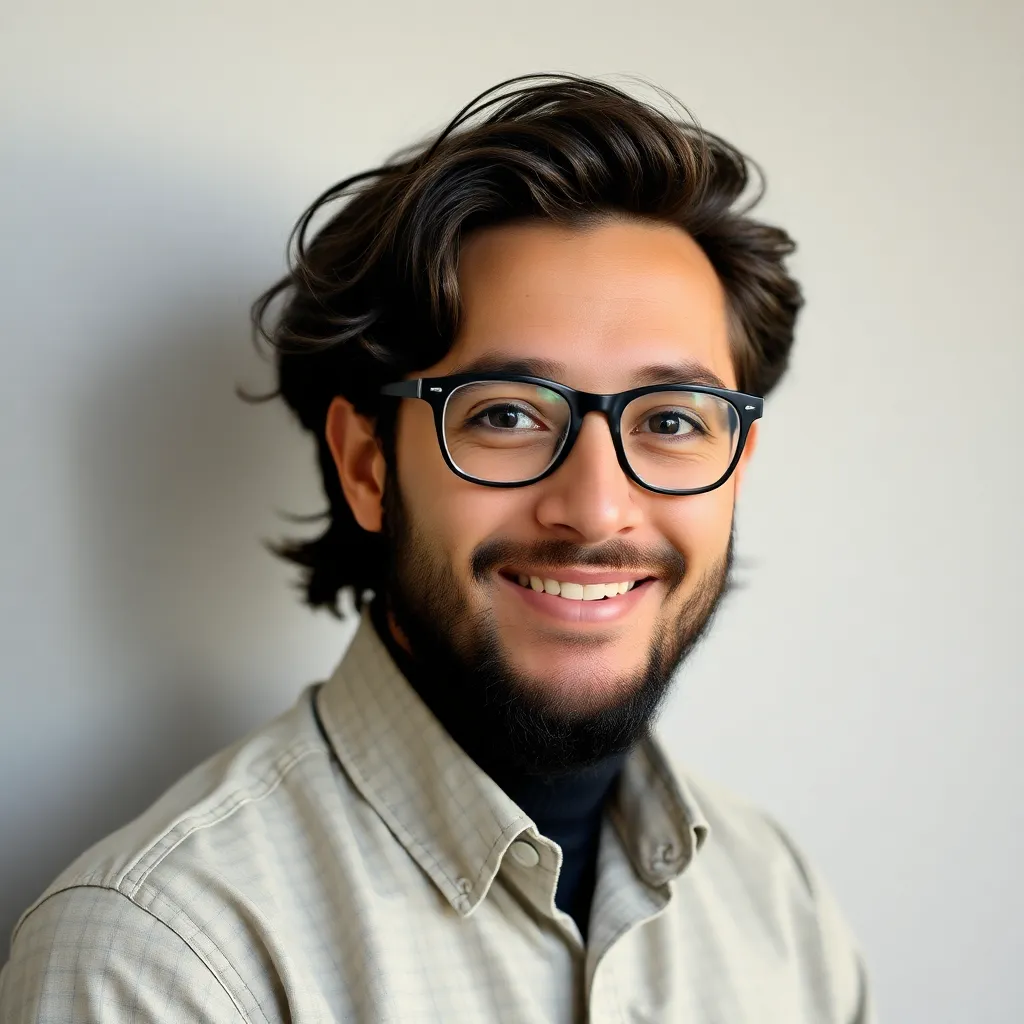
Muz Play
Apr 16, 2025 · 7 min read

Table of Contents
The Basic Building Blocks of the Nervous System: Neurons and Glia
The human nervous system, a marvel of biological engineering, is responsible for everything from our simplest reflexes to our most complex thoughts and emotions. This intricate network allows us to perceive the world, process information, and respond to our environment with remarkable speed and precision. At its foundation lie two fundamental cell types: neurons and glia. Understanding these basic building blocks is key to comprehending the complexities of the nervous system and the myriad neurological processes that shape our lives.
Neurons: The Communication Specialists
Neurons, the fundamental units of the nervous system, are specialized cells responsible for receiving, processing, and transmitting information. They achieve this through a complex interplay of electrical and chemical signals, enabling communication across vast networks within the brain and body. While neurons vary significantly in size and shape depending on their location and function, they share a common structural organization that facilitates this crucial communication role.
Key Structural Components of a Neuron:
-
Cell Body (Soma): The neuron's central hub, containing the nucleus and essential organelles responsible for maintaining cellular function. The soma integrates incoming signals and determines whether to initiate a signal of its own.
-
Dendrites: Branching extensions that emerge from the soma, acting like antennas to receive signals from other neurons. The surface area of dendrites is significantly increased by dendritic spines, tiny protrusions that form synapses with other neurons, increasing the neuron’s capacity for receiving and processing information. The more dendrites a neuron possesses, the more input it can receive, influencing the complexity of its processing.
-
Axon: A long, slender projection extending from the soma, specialized for transmitting signals over long distances. The axon's length can vary dramatically, from a few micrometers to over a meter in some cases, reflecting the neuron’s role in communication across different regions of the nervous system. The axon is covered in a myelin sheath (in many neurons), a fatty insulating layer that significantly speeds up signal transmission.
-
Axon Terminals (Synaptic Terminals): The branches at the end of the axon, forming specialized junctions called synapses with other neurons or target cells (e.g., muscle cells, gland cells). These terminals release neurotransmitters, chemical messengers that transmit signals to the next cell in the chain.
The Neuron's Electrical Signals: Action Potentials
Communication between neurons relies on electrical signals called action potentials. These are rapid, transient changes in the neuron's membrane potential – the voltage difference across its cell membrane. The process is as follows:
-
Resting Potential: In its resting state, the neuron maintains a negative membrane potential, typically around -70 millivolts (mV). This is due to a difference in ion concentration across the cell membrane, maintained by ion pumps and channels.
-
Depolarization: When a neuron receives sufficient stimulation, its membrane potential depolarizes – becomes less negative. If the depolarization reaches a threshold, it triggers an action potential.
-
Action Potential: A rapid, all-or-nothing electrical signal that propagates down the axon. The depolarization opens voltage-gated ion channels, allowing an influx of positively charged ions, primarily sodium (Na+), causing a dramatic reversal of the membrane potential.
-
Repolarization: Following the peak of the action potential, the membrane potential rapidly repolarizes, returning to its negative resting potential. This involves the outward flow of potassium (K+) ions.
-
Hyperpolarization: A brief period of hyperpolarization (membrane potential becomes more negative than the resting potential) follows repolarization, before the membrane potential gradually returns to its resting state. This refractory period prevents the immediate firing of another action potential, ensuring unidirectional signal propagation.
The speed of action potential propagation is influenced by factors such as axon diameter (larger axons conduct faster) and myelination (myelinated axons conduct much faster via saltatory conduction).
Neurotransmitters: Chemical Messengers
At the synapse, the action potential triggers the release of neurotransmitters from the presynaptic neuron's axon terminals. These neurotransmitters diffuse across the synaptic cleft, a tiny gap separating the presynaptic and postsynaptic neurons. They bind to specific receptors on the postsynaptic neuron's membrane, causing either excitation (depolarization, increasing the likelihood of an action potential) or inhibition (hyperpolarization, decreasing the likelihood of an action potential). The type of neurotransmitter and the type of receptor determine the effect on the postsynaptic neuron.
Glia: The Supporting Cast
While neurons are the stars of the nervous system's communication show, glial cells, often outnumbered by neurons, play a crucial supporting role. These cells are essential for maintaining the structural integrity of the nervous system, regulating the extracellular environment, and supporting neuronal function. There are several types of glia, each with distinct functions:
Astrocytes: The Multitaskers
Astrocytes are the most abundant type of glial cell in the brain. Their star-shaped morphology reflects their extensive branching and numerous contacts with both neurons and blood vessels. Their functions include:
-
Synaptic Support: Astrocytes regulate the synaptic environment, influencing neurotransmitter release and uptake. They also help form the blood-brain barrier, a protective barrier that limits the passage of substances from the bloodstream into the brain.
-
Metabolic Support: Astrocytes provide metabolic support to neurons, supplying them with nutrients and removing waste products. They are also involved in regulating ion homeostasis in the extracellular space, maintaining a stable neuronal environment.
-
Neuroprotection: Astrocytes protect neurons from damage, both from physical injury and from toxic substances. They play a role in the immune response within the central nervous system.
Oligodendrocytes and Schwann Cells: The Insulators
Oligodendrocytes (in the central nervous system) and Schwann cells (in the peripheral nervous system) are responsible for forming the myelin sheath that insulates axons. This myelin sheath significantly increases the speed of action potential propagation, allowing for rapid and efficient neuronal communication. Myelin is a fatty substance that wraps around the axon, creating segments of insulation interrupted by nodes of Ranvier. These nodes are crucial for saltatory conduction, a mechanism where the action potential "jumps" from node to node, dramatically increasing conduction speed.
Microglia: The Immune Defenders
Microglia are the resident immune cells of the central nervous system. They act as scavengers, removing cellular debris, pathogens, and damaged neurons. They also play a critical role in the brain's inflammatory response, responding to injury or infection. Microglia's role in both maintaining homeostasis and mediating inflammation is complex and essential for maintaining neuronal health.
Ependymal Cells: The CSF Producers
Ependymal cells line the ventricles of the brain and the central canal of the spinal cord. These cells are involved in the production and circulation of cerebrospinal fluid (CSF), a clear fluid that cushions and protects the brain and spinal cord. CSF is essential for maintaining the brain’s internal environment and for removing waste products.
The Interplay of Neurons and Glia: A Symphony of Function
The nervous system's remarkable complexity arises not only from the diversity of neurons but also from the intricate interplay between neurons and glia. Glial cells are not merely passive bystanders; they actively participate in shaping neuronal activity, influencing synaptic transmission, providing metabolic support, and protecting neurons from damage. Disruptions in glial function can have profound consequences on neuronal health and can contribute to the development of neurological disorders.
Examples of Neuron-Glia Interactions:
-
Synaptic Plasticity: Glial cells, particularly astrocytes, play a crucial role in synaptic plasticity, the ability of synapses to strengthen or weaken over time. This plasticity is fundamental for learning and memory.
-
Neuroinflammation: Microglia's response to injury or infection can trigger neuroinflammation, a process that can be both protective and harmful. While inflammation is necessary to clear away debris and pathogens, excessive inflammation can damage neurons and contribute to neurodegenerative diseases.
-
Myelin Formation and Maintenance: The proper formation and maintenance of the myelin sheath by oligodendrocytes and Schwann cells are crucial for efficient neuronal communication. Damage to myelin, as seen in multiple sclerosis, can lead to severe neurological deficits.
-
Blood-Brain Barrier Integrity: Astrocytes play a pivotal role in maintaining the integrity of the blood-brain barrier, which protects the brain from harmful substances in the bloodstream. Disruptions to this barrier can have serious consequences for brain health.
Understanding the basic building blocks of the nervous system – neurons and glia – and their intricate interactions is essential for advancing our knowledge of brain function and for developing effective treatments for neurological and psychiatric disorders. Continued research in this field promises to unlock further secrets of this incredible biological system and revolutionize our understanding of the mind and body's intricate connection. Further exploration into specific types of neurons, their neurotransmitters, and the diverse roles of glial cells will continue to unveil the complexity and elegance of the nervous system, driving progress in neuroscience and related fields. The ongoing research into the interaction between neurons and glia will continue to shape our understanding of health and disease within the nervous system, paving the way for more effective diagnostics and therapeutic strategies.
Latest Posts
Latest Posts
-
During His Trip On The Beagle Darwin Found That
Apr 19, 2025
-
Explain The Structure And Function Of The Respiratory Membrane
Apr 19, 2025
-
What Wavelength Does Chlorophyll A Absorb
Apr 19, 2025
-
What Could Happen Due To A Failure In Homeostasis
Apr 19, 2025
-
Fats Oils And Waxes Are All
Apr 19, 2025
Related Post
Thank you for visiting our website which covers about Basic Building Block Of The Nervous System . We hope the information provided has been useful to you. Feel free to contact us if you have any questions or need further assistance. See you next time and don't miss to bookmark.