Biologists Use The Fluid Mosaic Model To Describe Membrane Structure.
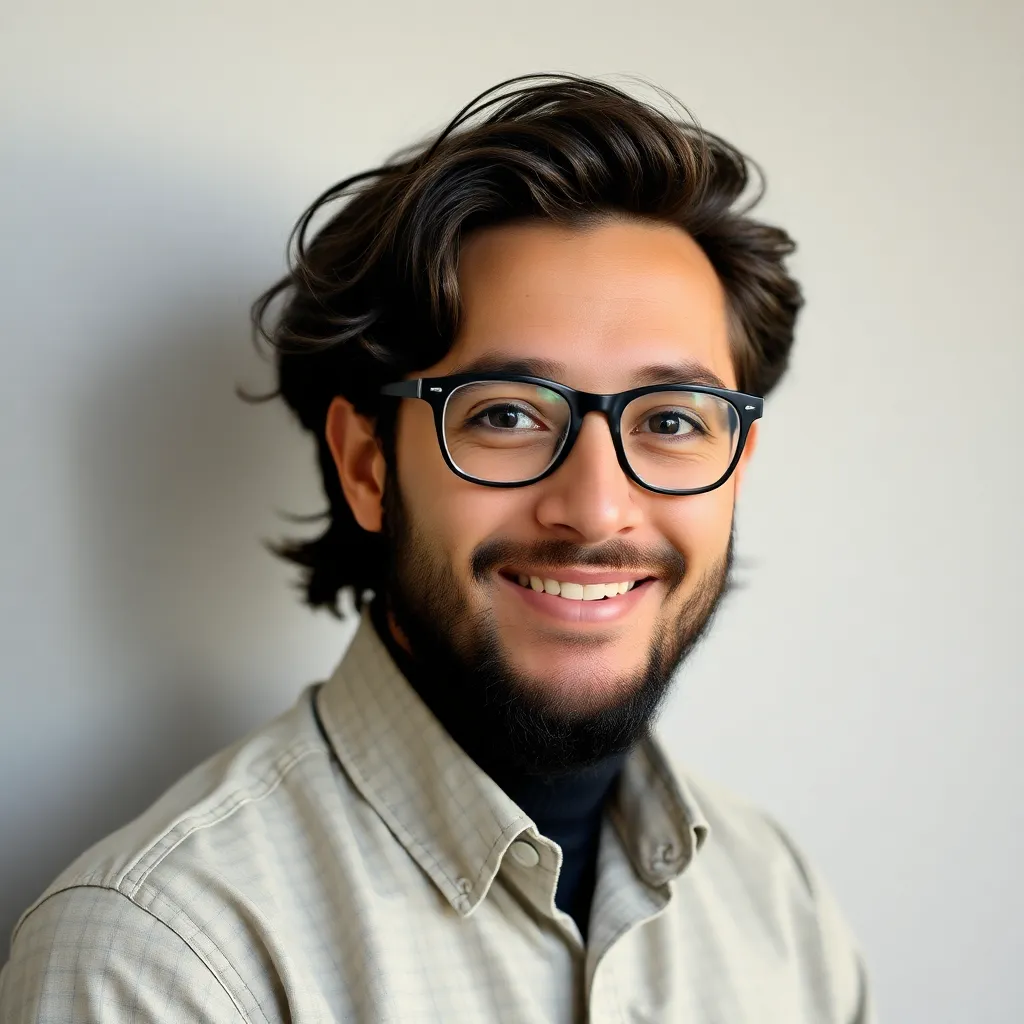
Muz Play
Apr 09, 2025 · 6 min read

Table of Contents
Biologists Use the Fluid Mosaic Model to Describe Membrane Structure
The cell membrane, a ubiquitous structure in all living organisms, is far more than just a simple barrier separating the inside of a cell from its external environment. It's a dynamic, complex, and exquisitely regulated interface that controls the passage of molecules, facilitates cell signaling, and plays a crucial role in maintaining cellular homeostasis. Biologists use the fluid mosaic model to describe this intricate structure, highlighting its fluidity and the diverse array of molecules embedded within it. This model, while continually refined with new discoveries, remains a cornerstone of cell biology understanding.
The Fluid Nature of the Membrane
The term "fluid" in the fluid mosaic model emphasizes the dynamic behavior of the membrane components. Instead of a rigid, static structure, the membrane is best visualized as a two-dimensional liquid, where the constituent molecules, primarily phospholipids, are constantly in motion. This fluidity is crucial for several cellular processes:
Lateral Diffusion: The Dance of Phospholipids
Phospholipids, the main structural components of the membrane, are amphipathic molecules, meaning they possess both hydrophilic (water-loving) and hydrophobic (water-fearing) regions. This characteristic leads to the formation of a bilayer, with the hydrophilic heads facing the aqueous environments inside and outside the cell, and the hydrophobic tails shielded within the core of the bilayer. These phospholipids aren't static; they constantly undergo lateral diffusion, moving sideways within their own leaflet (layer) of the bilayer. This lateral movement is rapid, allowing for membrane fluidity and flexibility.
Flip-Flop: A Less Frequent Movement
While lateral diffusion is common, the movement of a phospholipid from one leaflet to the other (transverse diffusion or "flip-flop") is far less frequent. This is because the hydrophilic head must traverse the hydrophobic core, an energetically unfavorable process. Specialized enzymes, called flippases, floppases, and scramblases, facilitate this flip-flop movement, playing crucial roles in maintaining membrane asymmetry and regulating lipid composition in each leaflet. This asymmetry is important for various cellular functions, including signal transduction and vesicle trafficking.
Factors Affecting Membrane Fluidity
The fluidity of the membrane isn't a constant; it's influenced by several factors:
- Temperature: Higher temperatures increase membrane fluidity, allowing for greater molecular movement. Conversely, lower temperatures decrease fluidity, potentially leading to a gel-like state.
- Fatty Acid Saturation: The degree of saturation of the fatty acid tails in phospholipids affects fluidity. Unsaturated fatty acids, with their kinks due to double bonds, prevent tight packing of phospholipids, increasing fluidity. Saturated fatty acids, lacking double bonds, pack more tightly, reducing fluidity.
- Cholesterol: Cholesterol, a crucial membrane component, acts as a fluidity buffer. At high temperatures, it reduces fluidity by restricting phospholipid movement. At low temperatures, it prevents the membrane from becoming too rigid by interfering with the packing of fatty acid tails. This role of cholesterol is particularly important in maintaining membrane integrity in organisms exposed to fluctuating temperatures.
The Mosaic Nature: A Diverse Array of Molecules
The "mosaic" aspect of the fluid mosaic model refers to the diverse range of molecules embedded within the phospholipid bilayer. This isn't a homogenous mixture; rather, these molecules are distributed heterogeneously, creating a complex and functional mosaic.
Membrane Proteins: The Workhorses of the Membrane
Membrane proteins are arguably the most important components of the mosaic. They perform a vast array of functions, including:
- Transport Proteins: These proteins facilitate the movement of ions and molecules across the membrane, either passively (e.g., channel proteins) or actively (e.g., pumps). They are critical for nutrient uptake, waste removal, and maintaining ion gradients.
- Receptor Proteins: These proteins bind to specific signaling molecules (ligands), triggering intracellular responses. This is vital for cell communication and signal transduction pathways.
- Enzymes: Many enzymes are embedded within the membrane, catalyzing reactions that occur at the membrane surface.
- Structural Proteins: These proteins provide structural support and maintain the integrity of the membrane. They anchor other membrane components and contribute to cell shape and adhesion.
- Glycoproteins and Glycolipids: Many membrane proteins and lipids are glycosylated, meaning they have carbohydrate chains attached. These carbohydrate moieties are involved in cell recognition, adhesion, and protection.
Protein Movement within the Membrane
Similar to phospholipids, membrane proteins can also move laterally within the membrane, though their movement might be restricted by interactions with the cytoskeleton or other membrane components. This lateral movement is crucial for dynamic processes such as receptor clustering and signal transduction.
Membrane Carbohydrates: The Cell's Identity Card
Carbohydrates, in the form of glycoproteins and glycolipids, are located on the outer surface of the membrane. These carbohydrate chains act as recognition sites for various molecules and cells, playing a critical role in cell-cell recognition, immune responses, and cell adhesion. They form a sort of "glycocalyx," a carbohydrate-rich layer surrounding the cell, which contributes to the cell's overall identity and interactions with its environment.
The Dynamic Nature of the Membrane: Adaptability and Response
The fluid mosaic model beautifully captures the dynamic nature of the cell membrane. Its fluidity allows the membrane to respond to changes in the environment, such as temperature fluctuations or ligand binding. This adaptability is essential for cellular survival and function.
Membrane Remodeling: A Constant Process
The membrane isn't a static structure; it's constantly undergoing remodeling. Phospholipids are synthesized, degraded, and exchanged, while proteins are inserted, removed, and laterally moved within the membrane. This constant remodeling allows the cell to adjust its membrane composition in response to changing needs and environmental stimuli.
Vesicle Trafficking: Membrane Dynamics in Action
The formation and fusion of vesicles, small membrane-bound sacs, are prime examples of membrane dynamics. Vesicles transport molecules within the cell, and their fusion with the plasma membrane allows for the secretion of substances and the uptake of materials via endocytosis. This vesicle trafficking underscores the fluid and adaptable nature of the membrane.
Beyond the Basic Model: Refinements and Ongoing Research
While the fluid mosaic model provides a fundamental understanding of membrane structure, ongoing research continues to refine our knowledge. For instance, the model is being expanded to incorporate more detailed information about:
- Lipid Rafts: These are microdomains within the membrane that are enriched in cholesterol and sphingolipids, creating more ordered and less fluid regions. They are thought to play a role in protein sorting, signal transduction, and endocytosis.
- Membrane Curvature: The membrane is not always flat; it can exhibit various curvatures, important for processes such as vesicle formation and membrane fusion. Specific proteins are involved in regulating membrane curvature.
- Membrane Asymmetry: The distinct composition of the inner and outer leaflets of the membrane is crucial for cellular functions, and the mechanisms maintaining this asymmetry are actively being investigated.
Conclusion: A Living, Breathing Structure
The fluid mosaic model is a powerful framework for understanding the complex structure and function of the cell membrane. It emphasizes the dynamic interplay of lipids and proteins, creating a fluid and adaptable structure that performs a multitude of essential cellular tasks. From regulating transport to facilitating communication, the cell membrane, as described by the fluid mosaic model, is a living, breathing structure, integral to the life of the cell and the organism as a whole. Ongoing research continues to illuminate the intricacies of this remarkable structure, revealing new layers of complexity and deepening our understanding of its crucial role in life's processes. Further investigation into the nuances of lipid rafts, protein interactions, and the dynamic regulation of membrane fluidity promises to further refine and enrich our comprehension of this fundamental biological component. The fluid mosaic model serves not just as a description, but as a dynamic framework for continued exploration and discovery within the realm of cell biology.
Latest Posts
Latest Posts
-
Difference Between Hypothesis Theory And Law Worksheet
Apr 17, 2025
-
Is Cutting Grass A Chemical Change
Apr 17, 2025
-
What Affects The Distribution Of Living Things On Earth
Apr 17, 2025
-
Are Ion Dipole Forces Stronger Than Hydrogen Bonds
Apr 17, 2025
-
Which Schedule Is The Most Resistant To Extinction
Apr 17, 2025
Related Post
Thank you for visiting our website which covers about Biologists Use The Fluid Mosaic Model To Describe Membrane Structure. . We hope the information provided has been useful to you. Feel free to contact us if you have any questions or need further assistance. See you next time and don't miss to bookmark.