Both Aerobic Respiration And Fermentation Begin With
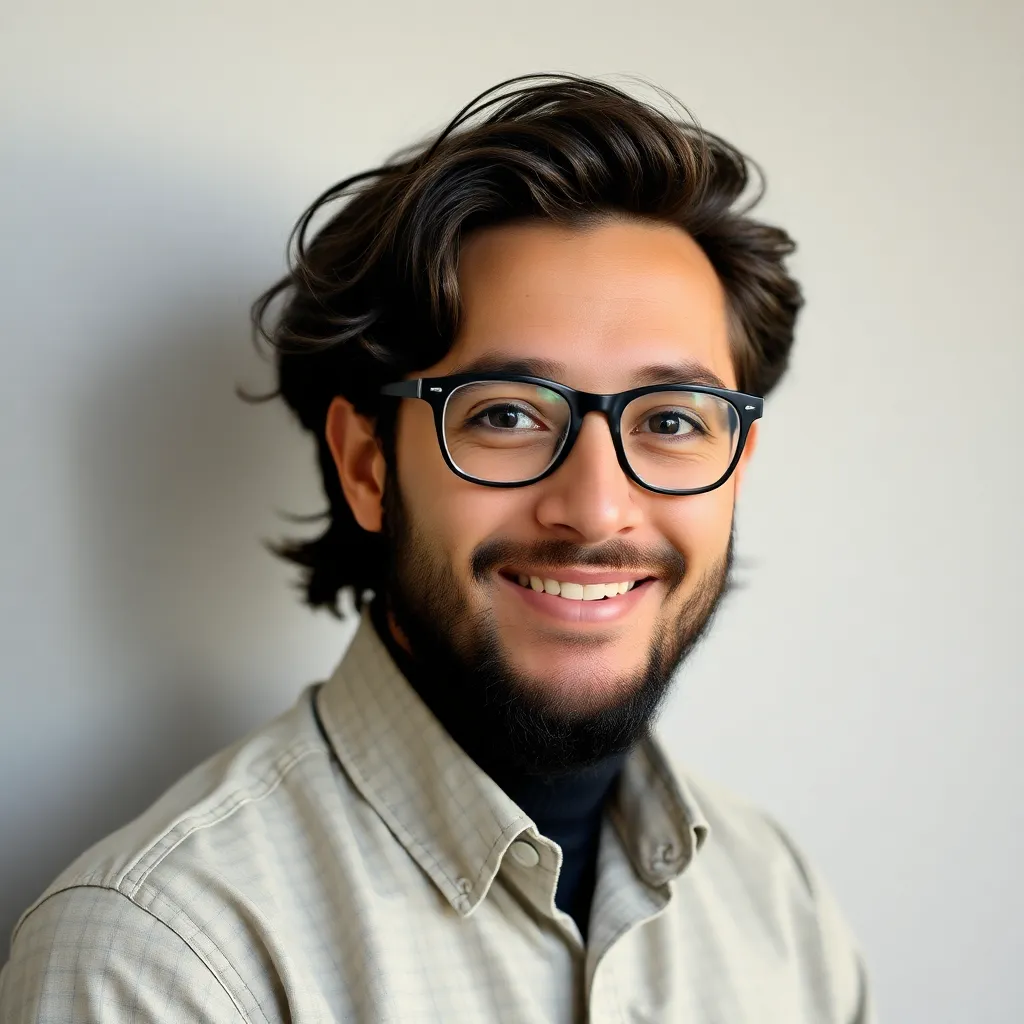
Muz Play
May 10, 2025 · 7 min read
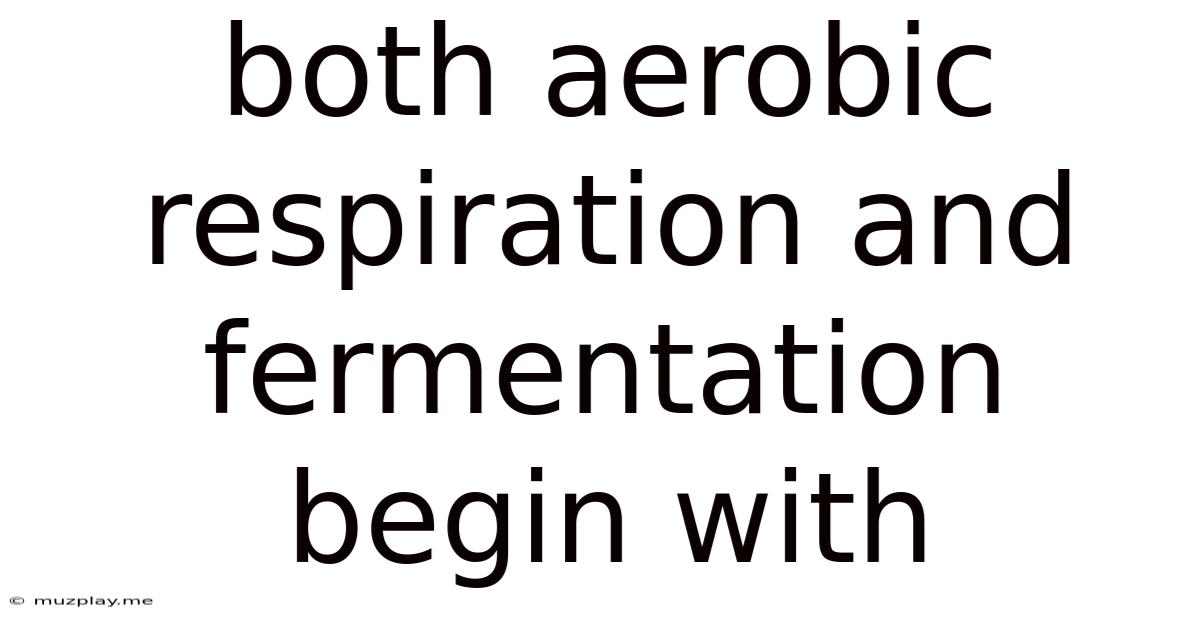
Table of Contents
Both Aerobic Respiration and Fermentation Begin With: Glycolysis – A Deep Dive into Cellular Energy Production
Cellular respiration is the fundamental process by which living organisms convert nutrients into energy, powering their diverse life functions. While vastly different in their mechanisms and end products, both aerobic respiration and fermentation share a crucial common starting point: glycolysis. This article delves deep into glycolysis, exploring its intricacies and explaining its pivotal role as the initial phase in both these crucial metabolic pathways. We will also examine the subsequent pathways unique to each process, highlighting the differences that dictate the efficiency of energy production.
Understanding Glycolysis: The Foundation of Energy Metabolism
Glycolysis, meaning "sugar splitting," is an anaerobic process – meaning it doesn't require oxygen – that occurs in the cytoplasm of all cells. It's a remarkably conserved pathway, suggesting its early evolution and fundamental importance to life. This ten-step process breaks down a single molecule of glucose (a six-carbon sugar) into two molecules of pyruvate (a three-carbon compound). Crucially, this breakdown generates a small, but significant, amount of ATP (adenosine triphosphate), the cell's primary energy currency, and NADH, a crucial electron carrier.
The Steps of Glycolysis: A Detailed Breakdown
Glycolysis can be broadly divided into two phases: the energy-investment phase and the energy-payoff phase.
1. Energy-Investment Phase (Steps 1-5): This initial phase requires an input of energy to prepare the glucose molecule for subsequent cleavage. Two ATP molecules are consumed during this phase, phosphorylating glucose and ultimately forming fructose-1,6-bisphosphate. This molecule is now unstable and ready to be split. Key enzymes involved include hexokinase, phosphoglucose isomerase, phosphofructokinase (a crucial regulatory enzyme), and aldolase.
2. Energy-Payoff Phase (Steps 6-10): This phase sees the breakdown of fructose-1,6-bisphosphate into two molecules of glyceraldehyde-3-phosphate (G3P). Through a series of oxidation and phosphorylation reactions, each G3P molecule yields two ATP molecules and one NADH molecule. This phase yields a net gain of 2 ATP and 2 NADH molecules per glucose molecule, considering the initial investment of 2 ATP. Important enzymes in this phase include glyceraldehyde-3-phosphate dehydrogenase, phosphoglycerate kinase, and pyruvate kinase.
The Importance of NADH: The Electron Shuttle
NADH (nicotinamide adenine dinucleotide) is a crucial coenzyme produced during glycolysis. It acts as an electron carrier, accepting high-energy electrons from the oxidation reactions in glycolysis. These electrons will be crucial in the subsequent stages of aerobic respiration or will be used in the alternative electron acceptors utilized during fermentation. The fate of NADH determines whether the cell proceeds with aerobic respiration or fermentation.
Aerobic Respiration: The Efficient Energy Pathway
If oxygen is available, pyruvate, the end product of glycolysis, enters the mitochondria, the powerhouse of the cell. Here, aerobic respiration continues in three major stages: the pyruvate oxidation, the Krebs cycle (or citric acid cycle), and the electron transport chain.
Pyruvate Oxidation: Transition to the Mitochondria
Pyruvate is transported across the mitochondrial membrane and undergoes oxidative decarboxylation. This process removes a carbon atom as carbon dioxide (CO2), releasing it as a waste product. The remaining two-carbon acetyl group is then attached to coenzyme A (CoA), forming acetyl-CoA. This step also produces one NADH molecule per pyruvate molecule (two per glucose).
The Krebs Cycle: A Cyclic Pathway of Oxidation
Acetyl-CoA enters the Krebs cycle, a series of enzymatic reactions that take place within the mitochondrial matrix. Through a cycle of oxidation and reduction reactions, the acetyl group is completely oxidized, releasing two molecules of CO2, one ATP, three NADH molecules, and one FADH2 molecule (another electron carrier) per acetyl-CoA molecule (thus, two ATP, six NADH, and two FADH2 per glucose). The Krebs cycle regenerates oxaloacetate, the initial substrate, ensuring continuous cycling.
The Electron Transport Chain: Oxidative Phosphorylation
The NADH and FADH2 molecules generated during glycolysis, pyruvate oxidation, and the Krebs cycle carry high-energy electrons to the electron transport chain (ETC) located in the inner mitochondrial membrane. Electrons are passed along a series of protein complexes, releasing energy at each step. This energy is used to pump protons (H+) across the inner mitochondrial membrane, creating a proton gradient.
This proton gradient drives ATP synthesis through chemiosmosis. Protons flow back across the membrane through ATP synthase, an enzyme that uses the energy of the proton gradient to phosphorylate ADP to ATP. This process, known as oxidative phosphorylation, is highly efficient, generating a substantial amount of ATP. Oxygen acts as the final electron acceptor in the ETC, combining with protons to form water (H2O).
Fermentation: An Anaerobic Alternative
In the absence of oxygen, cells resort to fermentation, an anaerobic process that allows glycolysis to continue. Fermentation doesn't produce ATP directly, but it regenerates NAD+ from NADH, enabling glycolysis to proceed and produce a small amount of ATP. There are several types of fermentation, including:
Lactic Acid Fermentation: Muscle Fatigue and Yogurt Production
In lactic acid fermentation, pyruvate is reduced directly to lactate, regenerating NAD+. This process occurs in muscle cells during strenuous exercise when oxygen supply is limited, leading to muscle fatigue. Lactic acid fermentation is also used in the production of yogurt and other fermented dairy products.
Alcoholic Fermentation: The Basis of Alcoholic Beverages
In alcoholic fermentation, pyruvate is first converted to acetaldehyde, releasing CO2. Acetaldehyde is then reduced to ethanol, regenerating NAD+. This process is used by yeast cells and is responsible for the production of alcoholic beverages and bread making. The CO2 produced during this process causes bread to rise.
Comparing Aerobic Respiration and Fermentation: Efficiency and Products
Aerobic respiration is far more efficient than fermentation in terms of ATP production. While glycolysis yields only 2 ATP molecules, aerobic respiration can produce significantly more—around 36-38 ATP molecules per glucose molecule. This substantial difference arises from the oxidative phosphorylation stage of aerobic respiration, which is absent in fermentation.
Feature | Aerobic Respiration | Fermentation |
---|---|---|
Oxygen Required | Yes | No |
ATP Production | 36-38 ATP per glucose molecule | 2 ATP per glucose molecule |
End Products | CO2, H2O | Lactate (lactic acid fermentation), Ethanol and CO2 (alcoholic fermentation) |
Location | Cytoplasm (glycolysis), Mitochondria | Cytoplasm |
Efficiency | High | Low |
The Significance of Glycolysis as the Universal Starter
The ubiquity of glycolysis across all domains of life underscores its fundamental role in energy metabolism. Its simplicity and efficiency make it an ideal starting point for both aerobic and anaerobic energy production. The pathway's regulation is tightly controlled, ensuring that cells can adapt to varying oxygen availability and energy demands. Understanding glycolysis is therefore crucial to comprehending the intricacies of cellular respiration and the remarkable adaptability of life.
Beyond Glucose: Alternative Fuel Sources
While glucose is the primary fuel for glycolysis, other sugars and molecules can also enter the pathway after undergoing conversion. For instance, fructose and galactose are metabolized into intermediates of glycolysis, allowing their energy to be harnessed. Other molecules, such as glycerol and amino acids, can also be converted into glycolytic intermediates, highlighting the versatility of this central metabolic pathway.
Regulatory Mechanisms of Glycolysis: A Fine-Tuned Process
The rate of glycolysis is precisely controlled by several regulatory mechanisms, ensuring that energy production matches cellular needs. Key regulatory enzymes, such as hexokinase and phosphofructokinase, are allosterically regulated by metabolites, such as ATP, ADP, and citrate. These regulatory mechanisms prevent excessive ATP production when energy is abundant and stimulate glycolysis when energy demand is high.
Clinical Significance: Glycolysis and Disease
Dysregulation of glycolysis is implicated in a variety of diseases, including cancer. Cancer cells often exhibit a heightened reliance on glycolysis, even in the presence of oxygen (the Warburg effect). This increased glycolytic activity provides cancer cells with the energy and building blocks needed for rapid growth and proliferation. Understanding the intricacies of glycolytic regulation is, therefore, crucial for developing new therapeutic strategies to target cancer cells.
Future Research Directions: Exploring the Glycolytic Landscape
Despite extensive research, many aspects of glycolysis remain under investigation. Researchers continue to explore the precise mechanisms of glycolytic regulation, the role of glycolysis in various diseases, and the potential for targeting glycolysis as a therapeutic strategy. Furthermore, ongoing research continues to reveal the intricate interactions between glycolysis and other metabolic pathways, offering further insights into the complex network of cellular metabolism.
In conclusion, glycolysis serves as the critical initial step in both aerobic respiration and fermentation, demonstrating its fundamental role in energy production for all living organisms. Understanding its intricacies, regulation, and implications for health and disease provides a crucial foundation for a deeper comprehension of cellular biology and metabolism. Its universal presence and versatile nature continue to fascinate and inspire researchers, promising further breakthroughs in our understanding of this vital metabolic pathway.
Latest Posts
Latest Posts
-
What Are The Tools Used To Measure Volume
May 11, 2025
-
Four Nitrogen Bases Found In Rna
May 11, 2025
-
What Is A Blank In A Spectrophotometer
May 11, 2025
-
What Lab Equipment Is Used To Measure Volume
May 11, 2025
-
Which Result Occurs During An Exothermic Reaction
May 11, 2025
Related Post
Thank you for visiting our website which covers about Both Aerobic Respiration And Fermentation Begin With . We hope the information provided has been useful to you. Feel free to contact us if you have any questions or need further assistance. See you next time and don't miss to bookmark.