Building Blocks Of The Nervous System
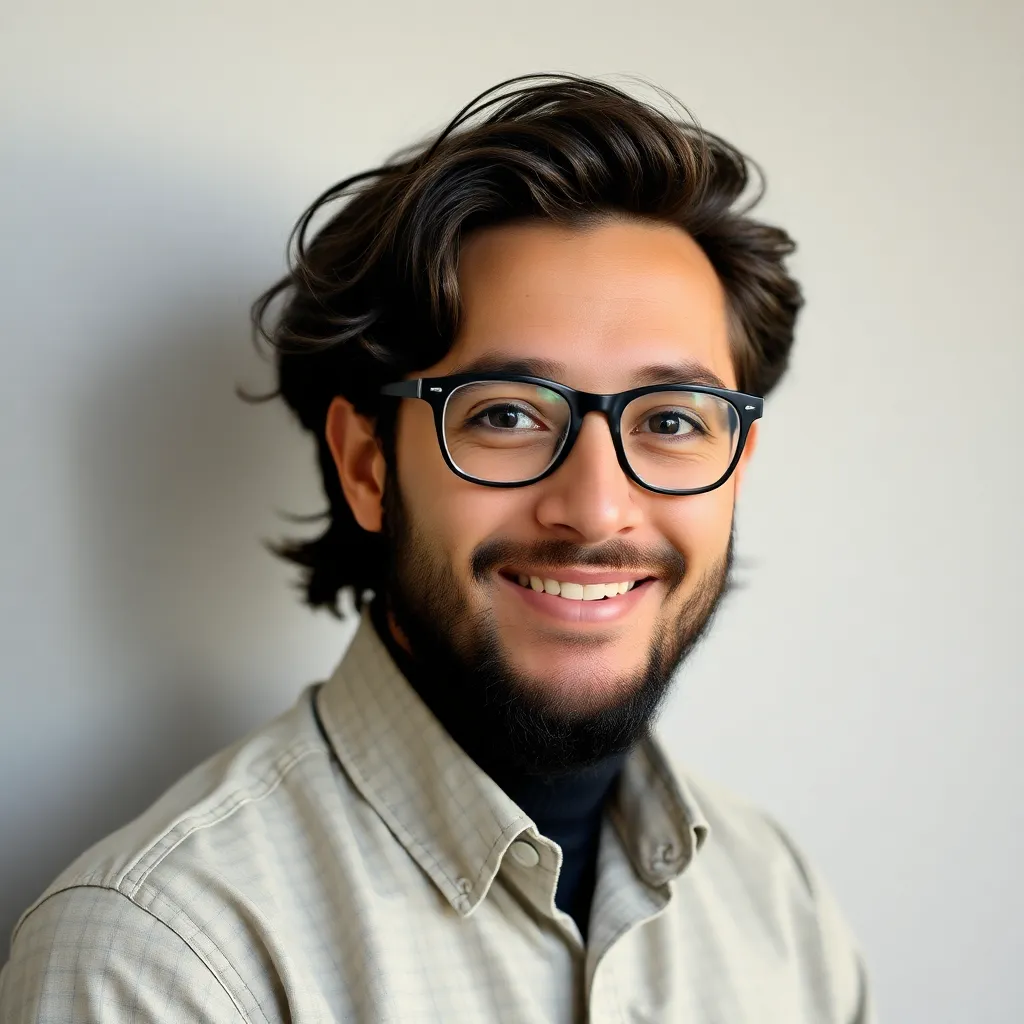
Muz Play
Apr 20, 2025 · 8 min read

Table of Contents
Building Blocks of the Nervous System: A Comprehensive Guide
The nervous system, a marvel of biological engineering, is the body's primary control center. It orchestrates everything from simple reflexes to complex thoughts and emotions. Understanding its intricate workings begins with grasping its fundamental building blocks: neurons and glial cells. This comprehensive guide delves into the structure, function, and interplay of these essential components, providing a solid foundation for appreciating the complexity and elegance of the nervous system.
I. Neurons: The Messengers of the Nervous System
Neurons, also known as nerve cells, are the fundamental units of the nervous system. They are specialized cells responsible for receiving, processing, and transmitting information throughout the body. Their ability to communicate rapidly and precisely is crucial for the coordinated function of all bodily systems.
A. Structure of a Neuron
A typical neuron possesses three main components:
-
Cell Body (Soma): The soma contains the nucleus and other essential organelles, like mitochondria, that are responsible for the neuron's metabolic processes. It's the neuron's control center, integrating incoming signals and initiating outgoing signals.
-
Dendrites: These are branching extensions of the soma that receive signals from other neurons. Their extensive branching significantly increases the surface area available for receiving input, allowing a single neuron to interact with numerous other neurons simultaneously. The more dendrites a neuron has, the more complex its integrative capacity. Dendritic spines, small protrusions on dendrites, further enhance the receiving surface area and play a role in synaptic plasticity.
-
Axon: A single, long projection extending from the soma. The axon transmits signals, known as action potentials, to other neurons, muscles, or glands. The axon's length can vary dramatically, from short connections within the brain to exceptionally long axons that extend from the spinal cord to the toes. Many axons are covered in a myelin sheath, a fatty insulating layer that significantly speeds up signal transmission. The gaps in the myelin sheath are called Nodes of Ranvier, and they play a crucial role in the process of saltatory conduction (explained below). At the end of the axon are axon terminals, also known as synaptic boutons or terminal buttons, which form synapses with other cells.
B. Types of Neurons
Neurons are incredibly diverse in terms of their size, shape, and function. They can be broadly classified based on their function:
-
Sensory Neurons (Afferent Neurons): These neurons transmit sensory information from the peripheral nervous system to the central nervous system (brain and spinal cord). They detect stimuli such as light, sound, touch, temperature, and pain, converting them into electrical signals that are relayed to the CNS.
-
Motor Neurons (Efferent Neurons): These neurons transmit signals from the CNS to muscles and glands, causing them to contract or secrete substances. They are responsible for voluntary movements, as well as the involuntary actions of the autonomic nervous system.
-
Interneurons: These neurons reside entirely within the CNS. They act as intermediaries, connecting sensory and motor neurons, and forming complex neural circuits that process information and generate appropriate responses. The vast majority of neurons in the brain are interneurons.
C. Neural Communication: The Action Potential
Neural communication relies on the rapid transmission of electrical signals known as action potentials. An action potential is a brief, all-or-nothing change in the electrical potential across the neuron's membrane. This process involves several key steps:
-
Resting Potential: In its resting state, the neuron maintains a negative electrical potential inside the cell relative to the outside. This potential difference is primarily due to the unequal distribution of ions (sodium, potassium, chloride) across the cell membrane.
-
Depolarization: When a stimulus reaches the neuron, it triggers the opening of voltage-gated sodium channels. Sodium ions rush into the cell, causing a rapid reversal of the membrane potential. This depolarization initiates the action potential.
-
Repolarization: Following depolarization, voltage-gated potassium channels open, allowing potassium ions to flow out of the cell. This restores the negative membrane potential.
-
Hyperpolarization: A brief period of hyperpolarization may occur, where the membrane potential becomes even more negative than the resting potential. This ensures that the neuron is refractory for a short period, preventing the backward propagation of the action potential.
-
Propagation: The action potential propagates down the axon, traveling as a wave of depolarization and repolarization. In myelinated axons, this propagation occurs through saltatory conduction, where the action potential "jumps" from one Node of Ranvier to the next.
D. Synaptic Transmission
The communication between neurons doesn't occur through direct contact. Instead, it happens through specialized junctions called synapses. At the synapse, the axon terminal of one neuron (the presynaptic neuron) releases neurotransmitters, chemical messengers that diffuse across the synaptic cleft (the gap between neurons) to bind to receptors on the dendrites or soma of the postsynaptic neuron.
This binding of neurotransmitters can either excite or inhibit the postsynaptic neuron, depending on the type of neurotransmitter and receptor. Excitatory neurotransmitters increase the likelihood of an action potential in the postsynaptic neuron, while inhibitory neurotransmitters decrease this likelihood. The integration of numerous excitatory and inhibitory signals determines whether the postsynaptic neuron will fire an action potential.
II. Glial Cells: The Supporting Cast of the Nervous System
While neurons are the stars of the nervous system show, glial cells play crucial supporting roles, ensuring the proper functioning of neurons and the overall health of the nervous system. Glial cells vastly outnumber neurons in the brain, performing a variety of essential tasks.
A. Types of Glial Cells
Several types of glial cells exist, each with its own specialized functions:
-
Astrocytes: These star-shaped cells are the most abundant glial cells in the brain. They provide structural support to neurons, regulate the extracellular environment (maintaining ion balance and neurotransmitter levels), and form the blood-brain barrier, which protects the brain from harmful substances in the bloodstream. Astrocytes also play a role in synaptic plasticity and neurotransmission.
-
Oligodendrocytes (CNS) and Schwann Cells (PNS): These cells produce the myelin sheath that insulates axons, significantly increasing the speed of signal transmission. Oligodendrocytes myelinate multiple axons in the central nervous system (brain and spinal cord), while Schwann cells myelinate a single axon in the peripheral nervous system.
-
Microglia: These are the immune cells of the nervous system. They act as scavengers, removing cellular debris, pathogens, and damaged neurons. They also play a role in inflammation and repair processes within the nervous system.
-
Ependymal Cells: These cells line the ventricles (fluid-filled cavities) of the brain and the central canal of the spinal cord. They produce and circulate cerebrospinal fluid (CSF), which cushions and protects the brain and spinal cord, and also transports nutrients and waste products.
B. Functions of Glial Cells
The roles of glial cells extend far beyond simple structural support. Their functions are integral to the overall health and functionality of the nervous system:
-
Structural Support: Glial cells provide physical scaffolding for neurons, holding them in place and maintaining the overall architecture of the nervous system.
-
Metabolic Support: Glial cells supply neurons with nutrients and remove metabolic waste products.
-
Insulation: Myelinating glial cells (oligodendrocytes and Schwann cells) greatly increase the speed of nerve impulse transmission.
-
Immune Defense: Microglia act as the immune cells of the nervous system, protecting against infection and injury.
-
Regulation of the Extracellular Environment: Astrocytes maintain the proper balance of ions and neurotransmitters in the extracellular space, crucial for neuronal function.
-
Blood-Brain Barrier: Astrocytes are essential components of the blood-brain barrier, which protects the brain from harmful substances in the bloodstream.
III. The Interplay between Neurons and Glial Cells
Neurons and glial cells don't function in isolation. They engage in a complex and dynamic interplay that is essential for the proper functioning of the nervous system. For instance:
-
Synaptic Plasticity: Astrocytes play a significant role in regulating synaptic strength and plasticity, the ability of synapses to strengthen or weaken over time. This process is crucial for learning and memory.
-
Neurotransmitter Uptake: Glial cells, particularly astrocytes, remove neurotransmitters from the synaptic cleft, terminating their action and preventing excessive stimulation or inhibition of the postsynaptic neuron.
-
Myelination: The myelin sheath produced by oligodendrocytes and Schwann cells is essential for efficient nerve impulse conduction. Damage to myelin, as seen in multiple sclerosis, can severely impair neural communication.
-
Neuroprotection: Glial cells, particularly microglia, protect neurons from damage and disease. They respond to injury or infection by removing cellular debris and promoting repair.
IV. Conclusion
The nervous system, with its astonishing complexity, is built upon the foundation of neurons and glial cells. These two fundamental cell types, although distinct in structure and function, work in concert to enable the intricate communication networks that underlie sensation, movement, thought, and emotion. Understanding their individual characteristics and their intricate interactions is paramount to comprehending the incredible power and vulnerability of the nervous system. Further research continues to unveil new facets of their roles and interactions, revealing the ongoing complexity and continuing mysteries of this vital system. The more we learn, the closer we get to understanding and addressing the challenges posed by neurological disorders and developing effective therapies for these debilitating conditions.
Latest Posts
Latest Posts
-
Which Proportionality Applies To Avogadros Law
Apr 20, 2025
-
How Does Respiratory System Help Maintain Homeostasis
Apr 20, 2025
-
Skin And Mucous Membranes Are Mostly Involved In
Apr 20, 2025
-
Are Acid Fast Negative Cells Stained By Carbolfuchsin
Apr 20, 2025
-
What Is The Principle Of Art
Apr 20, 2025
Related Post
Thank you for visiting our website which covers about Building Blocks Of The Nervous System . We hope the information provided has been useful to you. Feel free to contact us if you have any questions or need further assistance. See you next time and don't miss to bookmark.