Builds A New Dna Strand By Adding Complementary Bases
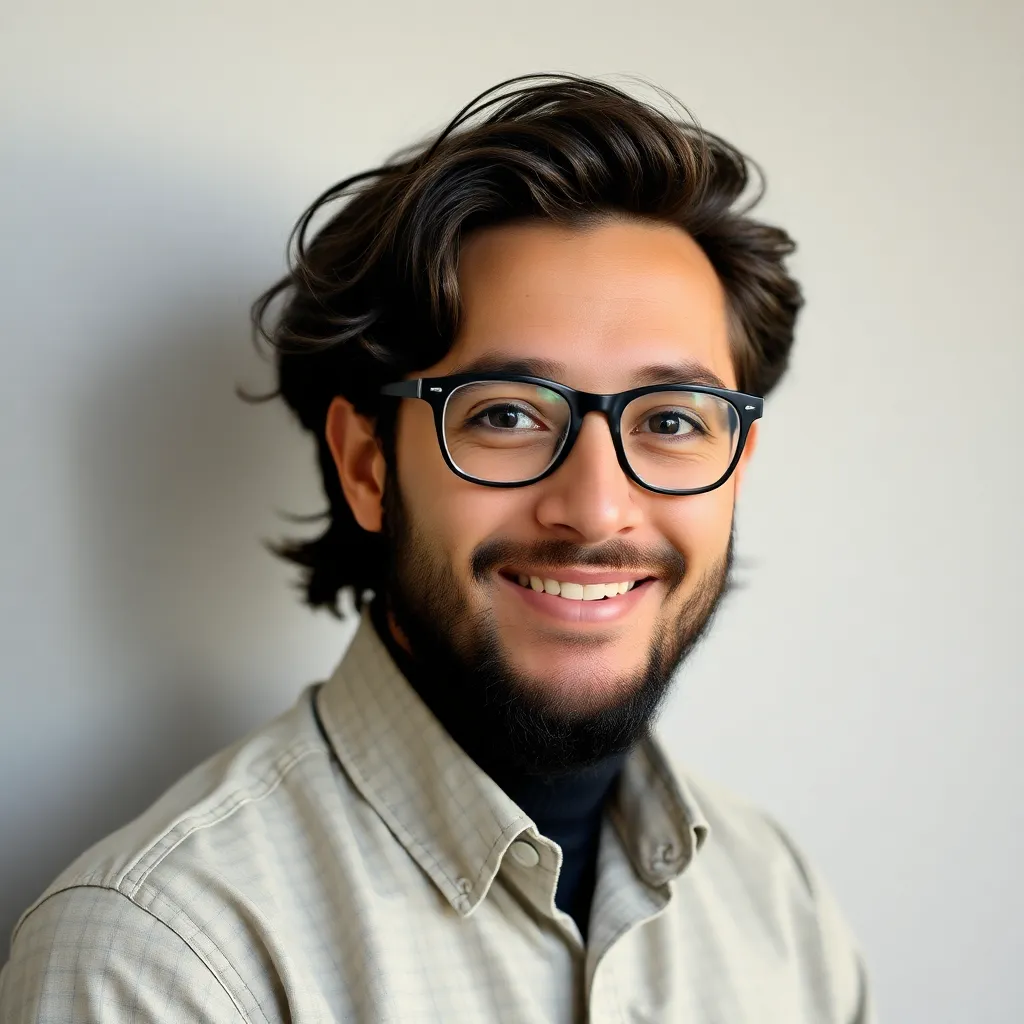
Muz Play
Apr 01, 2025 · 6 min read
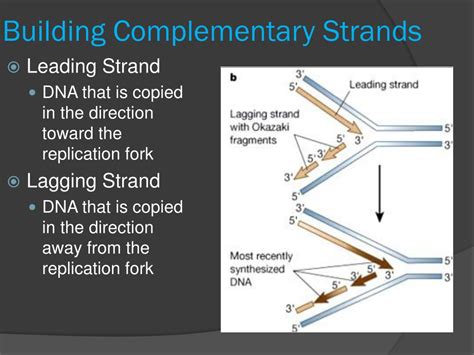
Table of Contents
Building a New DNA Strand: The Intricate Dance of Complementary Base Pairing
Deoxyribonucleic acid, or DNA, is the blueprint of life. This remarkable molecule holds the genetic instructions for the development, functioning, and reproduction of all known organisms and many viruses. Understanding how a new DNA strand is built, a process crucial for DNA replication and repair, is fundamental to comprehending the very essence of biology. This process relies heavily on the principle of complementary base pairing, a precise mechanism ensuring the fidelity of genetic information transfer.
The Double Helix and Complementary Base Pairing: The Foundation of DNA Replication
DNA's structure, a double helix resembling a twisted ladder, is intrinsically linked to its replication mechanism. The "rungs" of this ladder are formed by pairs of nitrogenous bases: adenine (A), thymine (T), guanine (G), and cytosine (C). Crucially, these bases pair specifically: adenine always pairs with thymine (A-T), and guanine always pairs with cytosine (G-C). This specific pairing, known as complementary base pairing, is the cornerstone of DNA replication.
Each strand of the DNA double helix acts as a template for the synthesis of a new, complementary strand. During replication, the double helix unwinds, separating the two parental strands. Each separated strand then serves as a template to direct the assembly of a new complementary strand. This process ensures that the genetic information is accurately copied, maintaining the integrity of the genome.
The Key Players: Enzymes and Proteins in DNA Replication
The process of building a new DNA strand isn't spontaneous; it's orchestrated by a complex molecular machinery involving several key enzymes and proteins. These components work in concert to ensure accurate and efficient DNA replication.
-
Helicase: This enzyme acts like a zipper, unwinding the DNA double helix at the replication fork, the point where the two strands separate. It breaks the hydrogen bonds holding the base pairs together, creating single-stranded templates.
-
Single-Strand Binding Proteins (SSBs): These proteins bind to the separated DNA strands, preventing them from reannealing (re-pairing) and keeping them stable for the incoming polymerase. They protect the single strands from damage and maintain the structure for effective replication.
-
Primase: DNA polymerase, the enzyme that adds new nucleotides, cannot initiate synthesis de novo. Primase solves this problem by synthesizing short RNA primers, providing a starting point for DNA polymerase. These primers are later removed and replaced with DNA.
-
DNA Polymerase: This is the central enzyme responsible for adding new nucleotides to the growing DNA strand. It reads the template strand and adds complementary nucleotides according to the base-pairing rules (A with T, and G with C). Different types of DNA polymerases have specific roles in replication, including proofreading to minimize errors.
-
Ligase: Once the RNA primers are removed and replaced with DNA, small gaps remain in the newly synthesized strand. Ligase seals these gaps, creating a continuous and complete DNA molecule.
-
Topoisomerase: As the DNA unwinds, it can create tension ahead of the replication fork. Topoisomerase relieves this tension by cutting and rejoining the DNA strands, preventing the formation of supercoils and ensuring smooth replication.
The Mechanism of DNA Strand Synthesis: A Detailed Look
The process of building a new DNA strand is highly regulated and involves several intricate steps:
-
Initiation: Replication begins at specific sites called origins of replication. These are specific DNA sequences where the helicase enzyme unwinds the double helix. Multiple origins of replication exist in eukaryotic chromosomes, allowing for faster replication of the large genomes.
-
Elongation: Once the DNA is unwound, primase synthesizes RNA primers. DNA polymerase then binds to the primers and begins adding complementary nucleotides to the 3' end of the growing strand. This is because DNA polymerase can only add nucleotides to the 3' hydroxyl group of the existing strand. This directionality is crucial to understanding the leading and lagging strands.
-
Leading Strand Synthesis: On one strand, the leading strand, synthesis proceeds continuously in the 5' to 3' direction, following the replication fork. A single RNA primer is sufficient to initiate continuous synthesis.
-
Lagging Strand Synthesis: On the other strand, the lagging strand, synthesis occurs discontinuously in short fragments called Okazaki fragments. Multiple RNA primers are needed, each initiating a new Okazaki fragment. These fragments are then joined together by ligase.
-
Termination: Replication terminates when the entire DNA molecule has been duplicated. Specific termination sequences signal the end of replication.
The Importance of Proofreading and Repair Mechanisms
DNA replication is remarkably accurate, but errors can still occur. Fortunately, DNA polymerase possesses proofreading activity. It can detect and correct mismatched bases, significantly reducing the error rate. Additional repair mechanisms exist to correct errors that escape the polymerase's proofreading. These mechanisms are vital for maintaining the integrity of the genome and preventing mutations that could lead to diseases.
Beyond Replication: DNA Strand Synthesis in Repair Processes
DNA strand synthesis is not limited to replication; it also plays a crucial role in DNA repair. Several DNA repair pathways involve the synthesis of new DNA strands to replace damaged or mutated segments. These pathways are crucial for maintaining genome stability and protecting the organism from harmful mutations.
Some examples of repair pathways involving DNA strand synthesis include:
-
Base Excision Repair (BER): This pathway targets damaged or modified bases. The damaged base is removed, and the resulting gap is filled by DNA polymerase using the undamaged strand as a template.
-
Nucleotide Excision Repair (NER): This pathway repairs bulky DNA lesions, such as those caused by UV radiation. A segment of the DNA containing the lesion is removed, and the gap is filled by DNA polymerase.
-
Mismatch Repair (MMR): This pathway corrects mismatched bases that escape the polymerase's proofreading. The mismatch is recognized, and the incorrect base is removed and replaced.
Clinical Significance: Errors in DNA Replication and Repair
Errors in DNA replication and repair can have significant consequences. Mutations arising from these errors can lead to various diseases, including cancer. Defects in DNA repair mechanisms can increase the risk of developing cancer and other genetic disorders. Understanding the mechanisms of DNA replication and repair is therefore crucial for developing therapies for these diseases.
The Future of DNA Strand Synthesis Research
Research into DNA strand synthesis continues to advance, with scientists exploring new techniques and technologies. These advances are leading to new insights into the intricate processes involved in DNA replication and repair, opening up exciting possibilities for applications in various fields. Understanding the mechanics of this process allows for the development of:
-
Improved gene editing technologies: CRISPR-Cas9 and other gene editing techniques rely on precise DNA strand synthesis for their effectiveness.
-
Advanced diagnostic tools: Understanding how errors in DNA synthesis contribute to disease can lead to better diagnostic tools and personalized medicine approaches.
-
Novel therapeutic strategies: Targeting specific aspects of DNA replication and repair could lead to the development of new cancer therapies and treatments for other genetic disorders.
In conclusion, building a new DNA strand by adding complementary bases is a fundamental process in all living organisms. The intricate interplay of enzymes, proteins, and the principle of complementary base pairing ensures accurate and efficient replication and repair of the genetic material. Continued research in this field promises further advancements with significant implications for medicine, biotechnology, and our understanding of life itself. The elegant precision of this molecular dance remains one of the most captivating marvels of the natural world.
Latest Posts
Latest Posts
-
How To Find Average Acceleration From Acceleration Time Graph
Apr 03, 2025
-
Who Is The First Person To See Cells
Apr 03, 2025
-
Which Of The Following Are Examples Of Homologous Structures
Apr 03, 2025
-
Which Plasma Component Is Not Present In Serum
Apr 03, 2025
-
Physical Features Map Of South America
Apr 03, 2025
Related Post
Thank you for visiting our website which covers about Builds A New Dna Strand By Adding Complementary Bases . We hope the information provided has been useful to you. Feel free to contact us if you have any questions or need further assistance. See you next time and don't miss to bookmark.