Cellular Respiration Releases Energy By Breaking Down What
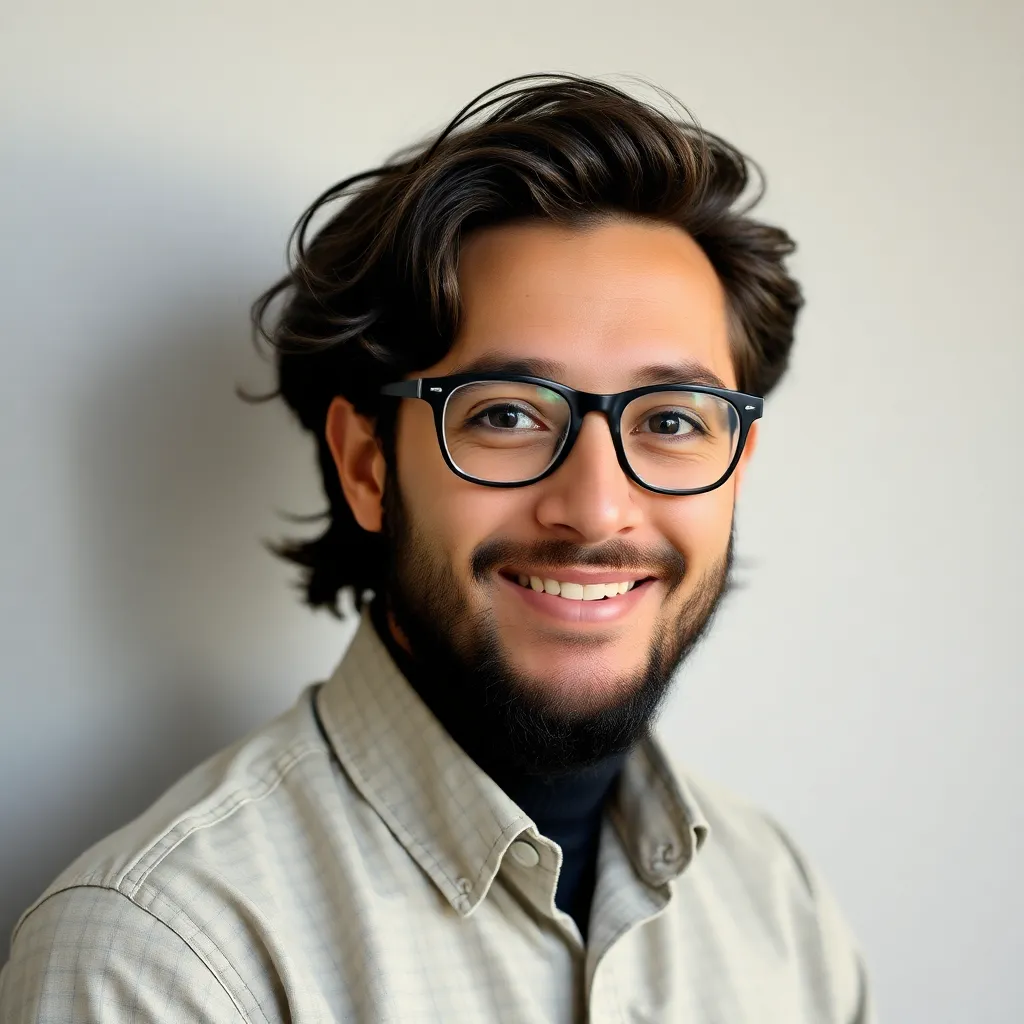
Muz Play
Apr 17, 2025 · 6 min read

Table of Contents
Cellular Respiration: Releasing Energy by Breaking Down Glucose
Cellular respiration is the fundamental process by which living organisms convert the chemical energy stored in organic molecules, primarily glucose, into a readily usable form of energy called ATP (adenosine triphosphate). This intricate process is essential for all life forms, providing the energy needed for a vast array of cellular functions, from muscle contraction and protein synthesis to nerve impulse transmission and maintaining cellular homeostasis. Understanding how cellular respiration releases energy by breaking down glucose is crucial to grasping the intricacies of life itself.
The Central Role of Glucose
Glucose, a simple sugar, acts as the primary fuel source for cellular respiration. It's a six-carbon molecule (C₆H₁₂O₆) packed with energy within its chemical bonds. This energy, however, isn't directly accessible to the cell. Cellular respiration acts as a sophisticated energy conversion system, carefully extracting energy from glucose in a controlled manner. The process isn't a single event, but rather a series of interconnected metabolic pathways that gradually release energy.
Glycolysis: The Initial Breakdown
The first stage of cellular respiration is glycolysis, meaning "sugar splitting". This anaerobic process (meaning it doesn't require oxygen) occurs in the cytoplasm of the cell. During glycolysis, one molecule of glucose is broken down into two molecules of pyruvate, a three-carbon compound. This breakdown releases a small amount of energy, directly producing a net gain of 2 ATP molecules and 2 NADH molecules. NADH is an electron carrier molecule that will play a crucial role in the subsequent stages of respiration. Glycolysis doesn't require oxygen, making it a crucial pathway for organisms that live in anaerobic environments.
The Transition to the Mitochondria: Pyruvate Oxidation
Following glycolysis, the pyruvate molecules are transported into the mitochondria, the cell's powerhouses. This process involves the conversion of pyruvate into acetyl-CoA, a two-carbon molecule. During this transition, carbon dioxide (CO₂) is released as a byproduct, and another molecule of NADH is produced. This step is crucial as it prepares the pyruvate for the energy-yielding reactions of the citric acid cycle.
The Citric Acid Cycle: A Central Metabolic Hub
The citric acid cycle (also known as the Krebs cycle or TCA cycle) takes place within the mitochondrial matrix. Here, acetyl-CoA enters a cyclical series of reactions, where it is systematically oxidized (loses electrons). Through a series of enzymatic reactions, the carbon atoms of acetyl-CoA are gradually released as carbon dioxide. This oxidation process releases a considerable amount of energy, which is captured in the form of ATP, NADH, and FADH₂, another electron carrier molecule. For each molecule of glucose (which yields two acetyl-CoA molecules), the citric acid cycle produces 2 ATP, 6 NADH, and 2 FADH₂.
The Importance of Electron Carriers
The NADH and FADH₂ molecules generated during glycolysis, pyruvate oxidation, and the citric acid cycle are crucial for the final stage of cellular respiration: oxidative phosphorylation. These molecules carry high-energy electrons, which will be used to drive the synthesis of ATP. They act as intermediaries, transferring electrons to the electron transport chain, the site of the major ATP production.
Oxidative Phosphorylation: The Major ATP Producer
Oxidative phosphorylation, the final and most energy-yielding stage of cellular respiration, occurs in the inner mitochondrial membrane. This process involves two main components: the electron transport chain (ETC) and chemiosmosis.
The Electron Transport Chain: A Cascade of Redox Reactions
The ETC consists of a series of protein complexes embedded in the inner mitochondrial membrane. Electrons from NADH and FADH₂ are passed along this chain through a series of redox reactions (reduction-oxidation reactions), where electrons are transferred from one molecule to another. Each transfer releases energy, which is used to pump protons (H⁺ ions) from the mitochondrial matrix across the inner membrane into the intermembrane space. This creates a proton gradient, with a higher concentration of protons in the intermembrane space than in the matrix.
Chemiosmosis: ATP Synthesis Driven by a Proton Gradient
The proton gradient established by the ETC creates a potential energy source. Protons flow back into the matrix through a protein complex called ATP synthase, a molecular turbine. This flow of protons drives the rotation of ATP synthase, which in turn catalyzes the synthesis of ATP from ADP (adenosine diphosphate) and inorganic phosphate (Pi). This process, called chemiosmosis, is responsible for the vast majority of ATP produced during cellular respiration. For each molecule of glucose, oxidative phosphorylation can yield approximately 32-34 ATP molecules.
The Overall Energy Yield of Cellular Respiration
In summary, the complete oxidation of one glucose molecule through cellular respiration yields a significant amount of ATP:
- Glycolysis: 2 ATP
- Citric Acid Cycle: 2 ATP
- Oxidative Phosphorylation: Approximately 32-34 ATP
This brings the total ATP yield to approximately 36-38 ATP molecules per glucose molecule. This is a highly efficient energy conversion process, providing the cell with the energy needed to fuel its diverse activities.
Variations in Cellular Respiration
While the core principles of cellular respiration remain consistent across different organisms, variations exist depending on the organism's metabolic needs and environmental conditions.
Anaerobic Respiration: Life Without Oxygen
In the absence of oxygen, some organisms can utilize alternative electron acceptors in place of oxygen during oxidative phosphorylation. This process, known as anaerobic respiration, allows them to generate ATP even in oxygen-deprived environments. Examples include fermentation pathways, such as lactic acid fermentation (in muscle cells) and alcoholic fermentation (in yeast). These pathways produce significantly less ATP than aerobic respiration.
Regulation of Cellular Respiration
Cellular respiration is a highly regulated process, ensuring that the production of ATP is matched to the cell's energy demands. Various factors, including the availability of glucose, oxygen levels, and the levels of ATP and ADP, influence the rate of respiration. Feedback mechanisms ensure that energy production is tightly controlled, preventing wasteful overproduction or energy deficiency.
The Significance of Cellular Respiration
Cellular respiration is not just a biochemical process; it's the engine of life. Its importance extends far beyond energy production:
-
Provides Energy for all Cellular Processes: From the basic functions like protein synthesis and cell division to complex activities like muscle contraction and nerve impulse transmission, cellular respiration powers them all.
-
Maintains Homeostasis: The energy generated sustains the intricate balance within the cell, maintaining cellular structure and function.
-
Supports Growth and Development: The energy released drives growth, repair, and development in organisms.
-
Essential for Life on Earth: Cellular respiration underpins the ecosystem by enabling the energy flow from producers (plants) to consumers (animals) and decomposers, supporting the entire web of life.
Conclusion: A Masterpiece of Biochemical Engineering
Cellular respiration stands as a testament to the elegance and efficiency of biological systems. This intricate process, where glucose is systematically broken down to release energy in a controlled manner, is fundamental to all life on Earth. By understanding the mechanisms of glycolysis, pyruvate oxidation, the citric acid cycle, and oxidative phosphorylation, we gain a deeper appreciation for the biochemical marvel that sustains life and shapes the world around us. Future research continues to uncover further details of this complex process, enhancing our understanding of cellular energy metabolism and its implications for health and disease. The ongoing study of cellular respiration is crucial for addressing numerous challenges, including developing new therapies for metabolic disorders and exploring alternative energy sources.
Latest Posts
Latest Posts
-
Indicate A Mild And Environmentally Safe Oxidizing Agent S
Apr 19, 2025
-
Which Of The Following Will Undergo Rearrangement Upon Heating
Apr 19, 2025
-
How To Find Density Of A Rock
Apr 19, 2025
-
What Process Is Used To Link Amino Acids Together
Apr 19, 2025
-
What Is The Mole Ratio Of Nh3 To N2
Apr 19, 2025
Related Post
Thank you for visiting our website which covers about Cellular Respiration Releases Energy By Breaking Down What . We hope the information provided has been useful to you. Feel free to contact us if you have any questions or need further assistance. See you next time and don't miss to bookmark.