Chair Conformation Of Alpha D Glucopyranose
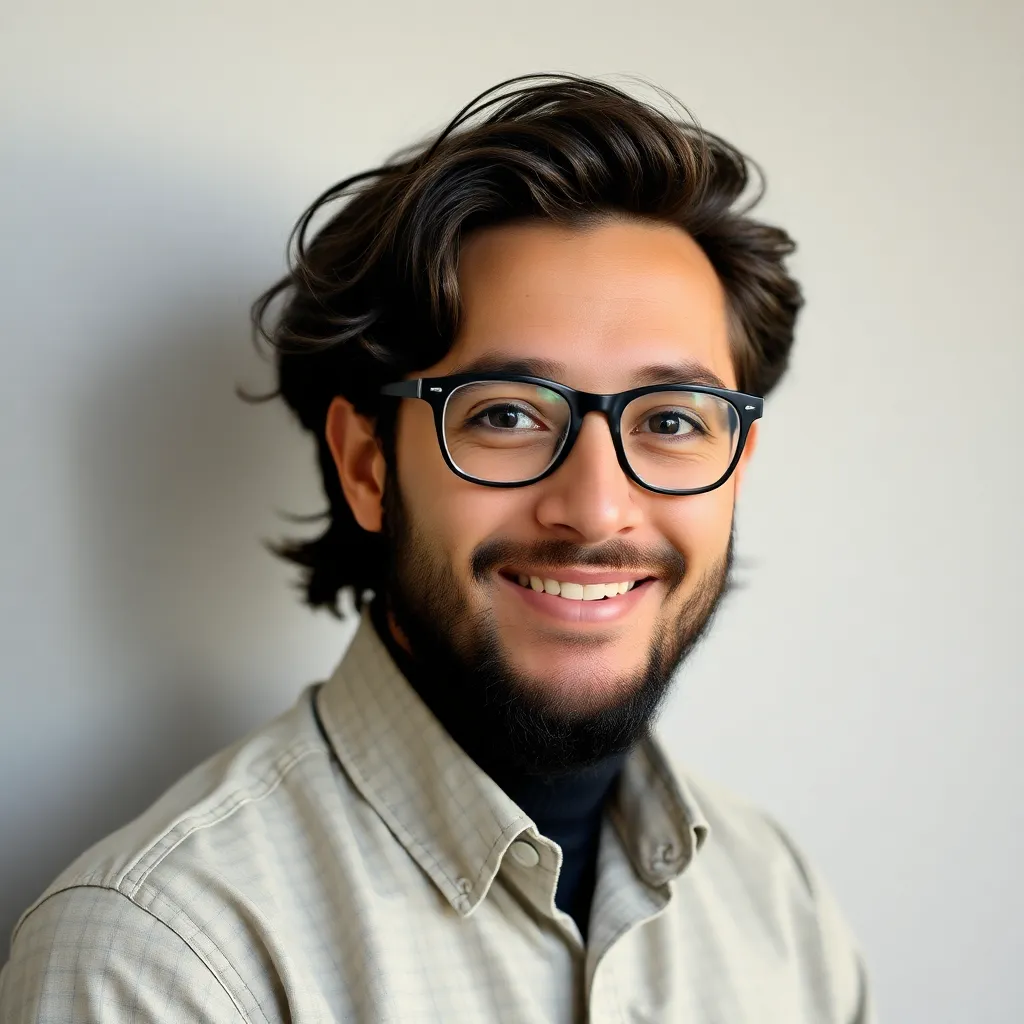
Muz Play
May 10, 2025 · 6 min read

Table of Contents
Chair Conformation of α-D-Glucopyranose: A Deep Dive
The world of carbohydrate chemistry can be intricate, with seemingly simple molecules exhibiting complex three-dimensional structures. Understanding these structures is crucial for comprehending their biological roles and reactivity. This article delves deep into the chair conformation of α-D-glucopyranose, a fundamental monosaccharide and a building block for many important biomolecules like starch and cellulose. We'll explore its conformational preferences, the factors influencing these preferences, and the implications for its chemical and biological properties.
Understanding the Basics: Pyranose Rings and Anomeric Carbon
Before we dissect the chair conformation, let's establish some fundamental concepts. Glucopyranose refers to the six-membered cyclic form of glucose, where the molecule forms a ring structure containing five carbon atoms and one oxygen atom. This ring is specifically a pyranose ring, resembling the heterocyclic compound pyran. The formation of this ring involves the reaction of the aldehyde group (C1) of glucose with the hydroxyl group (OH) on carbon 5. This reaction generates a new chiral center at C1, called the anomeric carbon. The two possible configurations of the hydroxyl group at the anomeric carbon are designated as α and β. This article focuses specifically on α-D-glucopyranose, where the hydroxyl group on the anomeric carbon is in the axial position (pointing down) in the chair conformation.
The Chair Conformation: Stability and Geometry
Cyclic sugars, like glucopyranose, do not exist as flat planar structures. Instead, they adopt a more stable three-dimensional conformation, predominantly the chair conformation. This is due to the reduction in steric strain and torsional strain compared to other possible conformations such as the boat or twist-boat conformations. In the chair conformation, the molecule adopts a puckered structure, resembling a chair.
The chair conformation has two types of substituents: axial and equatorial. Axial substituents are perpendicular to the plane of the ring, pointing directly up or down. Equatorial substituents lie roughly in the plane of the ring. The relative positions of these substituents dramatically impact the molecule's stability and reactivity.
Analyzing the α-D-Glucopyranose Chair Conformation
In the most stable chair conformation of α-D-glucopyranose, the following arrangement is observed:
- Anomeric hydroxyl group (OH at C1): Axial down. This is what defines it as the α-anomer.
- Hydroxyl group at C2: Equatorial down.
- Hydroxyl group at C3: Equatorial up.
- Hydroxyl group at C4: Equatorial down.
- Hydroxyl group at C6: Equatorial up (this is the CH<sub>2</sub>OH group, and its orientation is crucial for interactions).
This specific arrangement minimizes steric hindrance between the bulky substituents. If you were to draw the Haworth projection and then convert it to a chair conformation, carefully observing the orientation of each substituent (up or down) is critical for accurate representation.
Factors Influencing Chair Conformation Stability
Several factors contribute to the stability of the chair conformation of α-D-glucopyranose and the preference for this specific arrangement of substituents:
-
Steric hindrance: The bulkier the substituents, the greater the preference for equatorial positions to minimize steric clashes. Having hydroxyl groups in equatorial positions reduces the steric interactions between neighboring groups.
-
Anomeric effect: This is a significant factor influencing the conformation of glycosides. The anomeric effect describes the preference for the lone pair of electrons on the oxygen atom of the anomeric carbon to adopt an antiperiplanar orientation to the adjacent bond. In α-D-glucopyranose, the axial orientation of the anomeric hydroxyl group partially satisfies this preference, contributing to its stability despite the steric interactions.
-
Gauche effect: This effect describes the preference for gauche conformations in certain molecules, where atoms or groups are positioned at a dihedral angle of approximately 60 degrees. This plays a smaller role compared to steric and anomeric effects but still contributes to the overall stability.
-
Hydrogen bonding: Intramolecular and intermolecular hydrogen bonding can influence the overall stability of a conformation. The arrangement of hydroxyl groups in α-D-glucopyranose allows for favourable intramolecular hydrogen bonding interactions.
Comparing α-D-Glucopyranose with β-D-Glucopyranose
It's essential to compare α-D-glucopyranose with its isomer, β-D-glucopyranose. The key difference lies in the orientation of the hydroxyl group at the anomeric carbon (C1). In β-D-glucopyranose, this hydroxyl group is equatorial, resulting in a different arrangement of axial and equatorial substituents. While both conformations are stable, β-D-glucopyranose is generally slightly more stable due to the equatorial orientation of the anomeric hydroxyl group, which minimizes steric hindrance. However, the anomeric effect plays a significant role in stabilizing the α-anomer.
Implications for Biological Activity
The specific chair conformation of α-D-glucopyranose has significant implications for its biological functions. The precise arrangement of hydroxyl groups and their axial or equatorial orientations greatly influence the molecule's interactions with enzymes and other biomolecules. For instance:
-
Enzyme specificity: Enzymes often exhibit high specificity for their substrates. The chair conformation of α-D-glucopyranose, and the precise arrangement of its substituents, dictates which enzymes can effectively bind to and catalyze reactions involving this molecule. This is crucial in metabolic processes, like the digestion of starch and cellulose.
-
Glycosidic bond formation: The reactivity of the anomeric carbon is heavily influenced by its axial orientation in α-D-glucopyranose. This affects how it participates in glycosidic bond formation, linking monosaccharides to create disaccharides, oligosaccharides, and polysaccharides. The orientation of the anomeric hydroxyl group determines the configuration of the glycosidic linkage (α or β).
-
Intermolecular interactions: The specific positioning of the hydroxyl groups also impacts how α-D-glucopyranose interacts with other molecules through hydrogen bonding. This is vital for the formation of complex structures like starch granules and cellulose microfibrils.
Methods for Studying Chair Conformation
Several techniques are employed to study and confirm the chair conformation of α-D-glucopyranose:
-
X-ray crystallography: This technique allows for the determination of the three-dimensional structure of molecules in the solid state.
-
NMR spectroscopy: Nuclear magnetic resonance (NMR) spectroscopy is a powerful technique for determining the conformation of molecules in solution. Specific NMR parameters, such as coupling constants, can provide information about the dihedral angles between atoms, indicating whether substituents are axial or equatorial.
-
Computational methods: Molecular modeling and computational simulations can be used to predict and optimize the structure of α-D-glucopyranose, providing insights into its conformational preferences and energy differences between different conformations.
Conclusion: A Crucial Conformational Detail
The chair conformation of α-D-glucopyranose is not simply a structural detail; it's a critical factor determining the molecule's reactivity and biological function. Understanding the interplay of steric hindrance, the anomeric effect, and other factors influencing this conformation is crucial for appreciating the complexity of carbohydrate chemistry and the pivotal role of this simple sugar in biological systems. This intricate structure, seemingly simple at first glance, highlights the remarkable precision of nature's design and the importance of understanding three-dimensional structures at a molecular level. Further research continues to unravel the finer details of α-D-glucopyranose's conformational preferences and their implications for various biological processes, highlighting the ongoing relevance and significance of this molecule.
Latest Posts
Latest Posts
-
Not A One To One Function
May 10, 2025
-
Does Bbr3 Obey The Octet Rule
May 10, 2025
-
Select Ways In Which Animals Incorporate Phosphate Into Their Bodies
May 10, 2025
-
Alcohol De Maiz Para Que Sirve
May 10, 2025
-
Cu Ag And Au Are All In What Group
May 10, 2025
Related Post
Thank you for visiting our website which covers about Chair Conformation Of Alpha D Glucopyranose . We hope the information provided has been useful to you. Feel free to contact us if you have any questions or need further assistance. See you next time and don't miss to bookmark.