Change In Allele Frequency Over Time
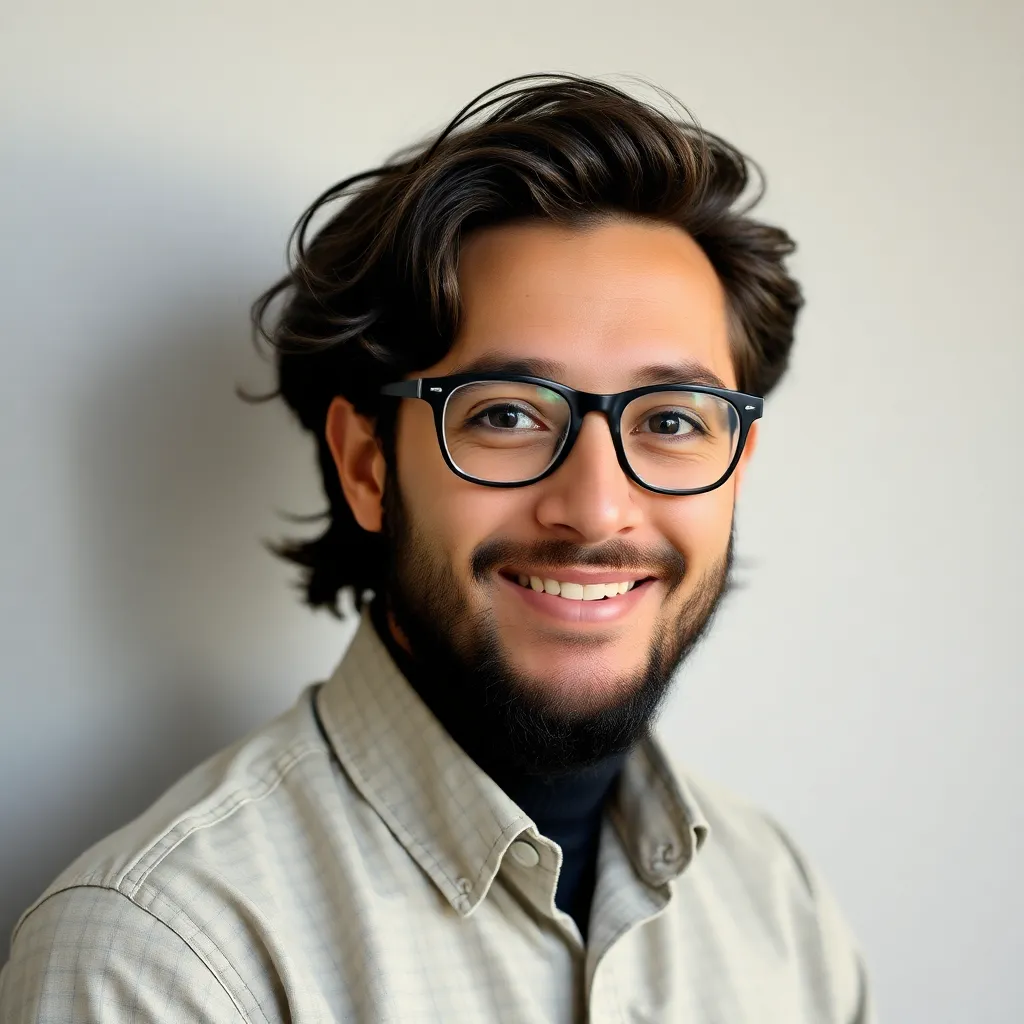
Muz Play
Apr 06, 2025 · 7 min read
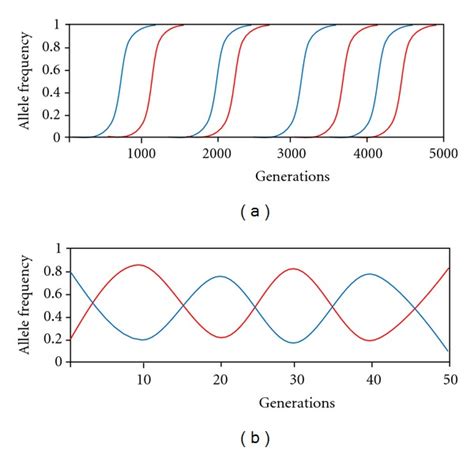
Table of Contents
Change in Allele Frequency Over Time: A Deep Dive into Population Genetics
The study of how allele frequencies change within a population over time is a cornerstone of population genetics. This fascinating field delves into the mechanisms that drive evolution, offering insights into the diversity of life on Earth and the processes that shape it. Understanding these changes is crucial for comprehending everything from the emergence of new species to the prevalence of genetic diseases. This article will explore the various factors influencing allele frequency shifts, including natural selection, genetic drift, mutation, gene flow, and non-random mating.
What are Alleles and Allele Frequencies?
Before diving into the dynamics of change, let's establish a clear understanding of the fundamental concepts. Alleles are different versions of the same gene. For instance, a gene for eye color might have alleles for brown eyes and blue eyes. Within a population, the allele frequency represents the proportion of a specific allele among all alleles for that particular gene. For example, if in a population of 100 individuals, 60 have the allele for brown eyes and 40 have the allele for blue eyes, the allele frequency for the brown eye allele is 0.6 (60/100), and the frequency for the blue eye allele is 0.4 (40/100). These frequencies always add up to 1 (or 100%).
Mechanisms Driving Changes in Allele Frequencies
Several forces can significantly alter allele frequencies over time, often interacting in complex ways. Let's examine each in detail:
1. Natural Selection: The Driving Force of Adaptation
Natural selection is arguably the most influential mechanism driving changes in allele frequencies. It favors alleles that enhance an organism's survival and reproductive success in a given environment. Individuals carrying advantageous alleles are more likely to survive and reproduce, passing on those beneficial alleles to their offspring. Over generations, the frequency of these advantageous alleles increases within the population, while less advantageous alleles decrease. This process leads to adaptation—the gradual improvement of a population's fit to its environment.
Examples of Natural Selection:
- Peppered moths: The classic example illustrates how environmental changes can dramatically shift allele frequencies. During the Industrial Revolution, pollution darkened tree bark, making darker moths better camouflaged against predators. The frequency of the allele for dark coloration increased significantly.
- Antibiotic resistance: The overuse of antibiotics has driven the evolution of antibiotic-resistant bacteria. Bacteria with alleles conferring resistance survive antibiotic treatment and reproduce, leading to a rapid increase in the frequency of resistance alleles within bacterial populations.
- Sickle cell anemia: In regions with high malaria prevalence, individuals carrying one copy of the sickle cell allele have increased resistance to malaria. This heterozygote advantage maintains a relatively high frequency of the sickle cell allele, even though individuals with two copies suffer from the debilitating disease.
2. Genetic Drift: Random Fluctuations in Allele Frequencies
Unlike natural selection, genetic drift is a random process that can significantly alter allele frequencies, especially in small populations. It arises from chance events, such as random sampling of gametes during reproduction or random fluctuations in survival and reproduction. These random fluctuations can lead to the loss of alleles, even advantageous ones, or the fixation of alleles, even detrimental ones.
Types of Genetic Drift:
- Bottleneck effect: A severe reduction in population size, often due to a catastrophic event, can dramatically alter allele frequencies. The surviving individuals may not represent the genetic diversity of the original population, resulting in a loss of alleles.
- Founder effect: When a small group of individuals colonizes a new area, the allele frequencies in the founding population may differ significantly from the original population. This can lead to the establishment of unique allele frequencies in the new population.
3. Mutation: The Ultimate Source of New Alleles
Mutations are changes in the DNA sequence that can create new alleles. While mutations are generally rare, they are the ultimate source of genetic variation within a population. Most mutations are neutral or harmful, but some can be beneficial, providing the raw material for natural selection to act upon. The rate of mutation is typically low, but over long periods, it can significantly contribute to changes in allele frequencies.
4. Gene Flow: The Movement of Alleles Between Populations
Gene flow refers to the movement of alleles between populations through migration. Individuals migrating from one population to another can introduce new alleles or alter the frequencies of existing alleles in the recipient population. Gene flow can counteract the effects of natural selection and genetic drift, promoting homogeneity between populations.
5. Non-random Mating: Assortative and Disassortative Mating
Non-random mating occurs when individuals do not choose mates randomly. Two major types exist:
- Assortative mating: Individuals with similar phenotypes mate more frequently than expected by chance. This can increase the homozygosity (two identical alleles) for certain genes.
- Disassortative mating: Individuals with dissimilar phenotypes mate more frequently. This increases heterozygosity (two different alleles).
Both types of non-random mating can affect allele frequencies over time, although they don't directly introduce new alleles or remove existing ones like the other mechanisms.
The Hardy-Weinberg Principle: A Null Model
The Hardy-Weinberg principle provides a null model for understanding allele frequency changes. It states that in the absence of evolutionary forces (natural selection, genetic drift, mutation, gene flow, and non-random mating), allele and genotype frequencies will remain constant from one generation to the next. This principle provides a baseline against which to compare real-world populations and identify the influence of evolutionary forces. The Hardy-Weinberg equilibrium is rarely observed in natural populations, making it a valuable tool for detecting evolutionary change.
Measuring Changes in Allele Frequencies: Quantitative Approaches
Several methods can quantify changes in allele frequencies over time. These often involve statistical analysis of population data, including:
- Allele frequency calculations: Direct calculation of allele frequencies from genotypic data.
- Hardy-Weinberg equilibrium tests: Statistical tests to determine whether a population deviates from Hardy-Weinberg equilibrium, suggesting the action of evolutionary forces.
- Phylogenetic analyses: Tracing allele evolution across different populations or species to reconstruct evolutionary history and identify changes in allele frequencies over time.
- Population genetic simulations: Computer models can simulate the effects of various evolutionary forces on allele frequencies, allowing researchers to test hypotheses and make predictions.
Applications of Understanding Allele Frequency Changes
The study of allele frequency changes has far-reaching applications in various fields:
- Conservation biology: Understanding how allele frequencies change in endangered species is crucial for developing effective conservation strategies. Maintaining genetic diversity is essential for the long-term survival of populations.
- Medicine: Tracking changes in allele frequencies associated with disease susceptibility can help identify genetic risk factors and develop targeted therapies. Understanding the evolution of antibiotic resistance is also critical for developing effective treatments.
- Agriculture: Monitoring allele frequencies in crop and livestock populations can help improve breeding programs and enhance productivity.
- Forensic science: Allele frequencies are used in DNA fingerprinting and paternity testing.
- Anthropology: Studying allele frequency changes in human populations helps understand human migration patterns, evolutionary history, and relationships between different groups.
Conclusion: The Dynamic Landscape of Allele Frequencies
Changes in allele frequencies are the driving force of evolution. The interplay of natural selection, genetic drift, mutation, gene flow, and non-random mating shapes the genetic composition of populations over time. Understanding these mechanisms is critical for unraveling the complexities of life's diversity and for addressing many pressing challenges facing humanity, from conservation efforts to medical advancements. Ongoing research continues to refine our understanding of these processes, providing new insights into the fascinating dynamics of allele frequencies and their role in shaping the evolutionary landscape. By utilizing sophisticated statistical and computational methods, researchers are able to further our understanding of this fundamental aspect of life on Earth, enriching our knowledge of both past events and future possibilities. The continued study of allele frequency change promises exciting discoveries in the years to come.
Latest Posts
Latest Posts
-
How To Tell If A Transformation Is Linear
Apr 06, 2025
-
How To Find The Kernel Of A Linear Transformation
Apr 06, 2025
-
Examples Of Strong And Weak Bases
Apr 06, 2025
-
A Surface Encumbrance Is Anything That Could Create A
Apr 06, 2025
-
Do Protists Make Their Own Food
Apr 06, 2025
Related Post
Thank you for visiting our website which covers about Change In Allele Frequency Over Time . We hope the information provided has been useful to you. Feel free to contact us if you have any questions or need further assistance. See you next time and don't miss to bookmark.