Choose When Quenching Occurs In A Chemiluminescence Reaction
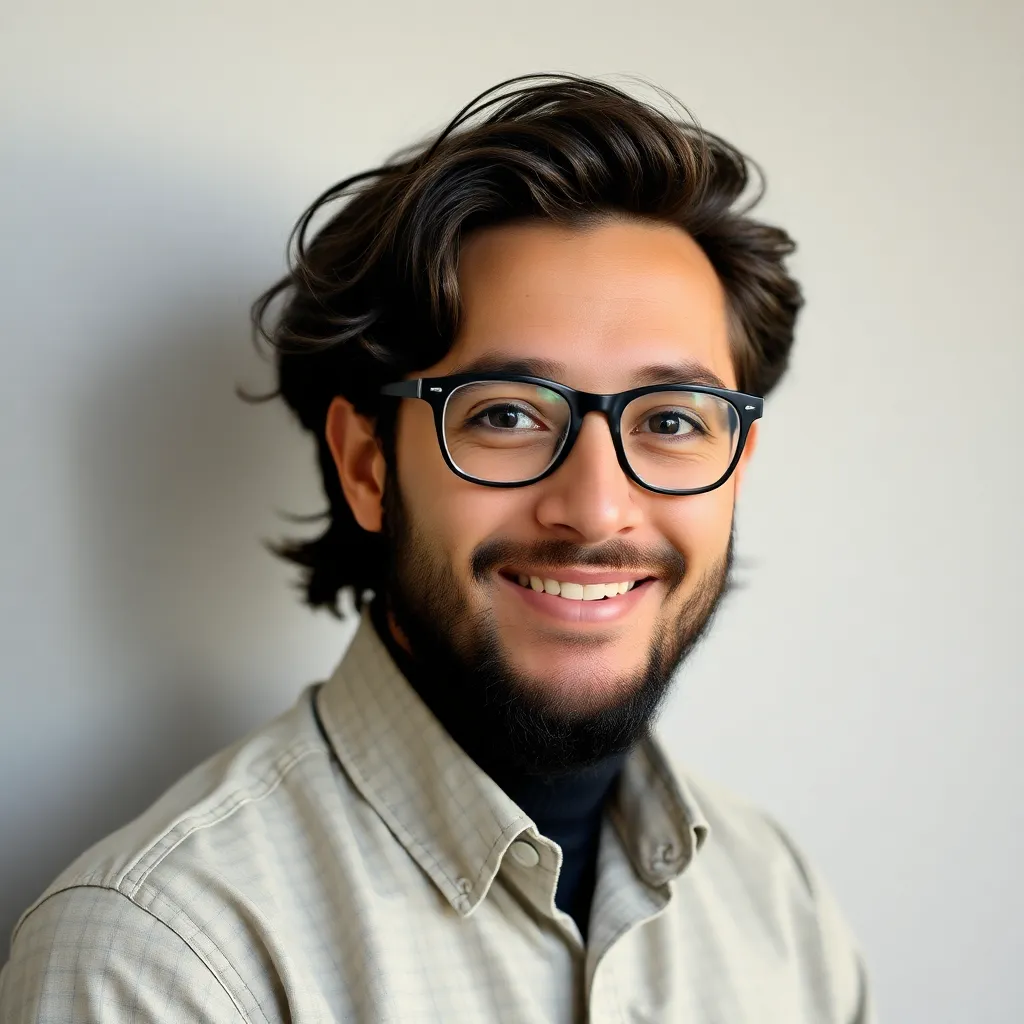
Muz Play
May 10, 2025 · 7 min read
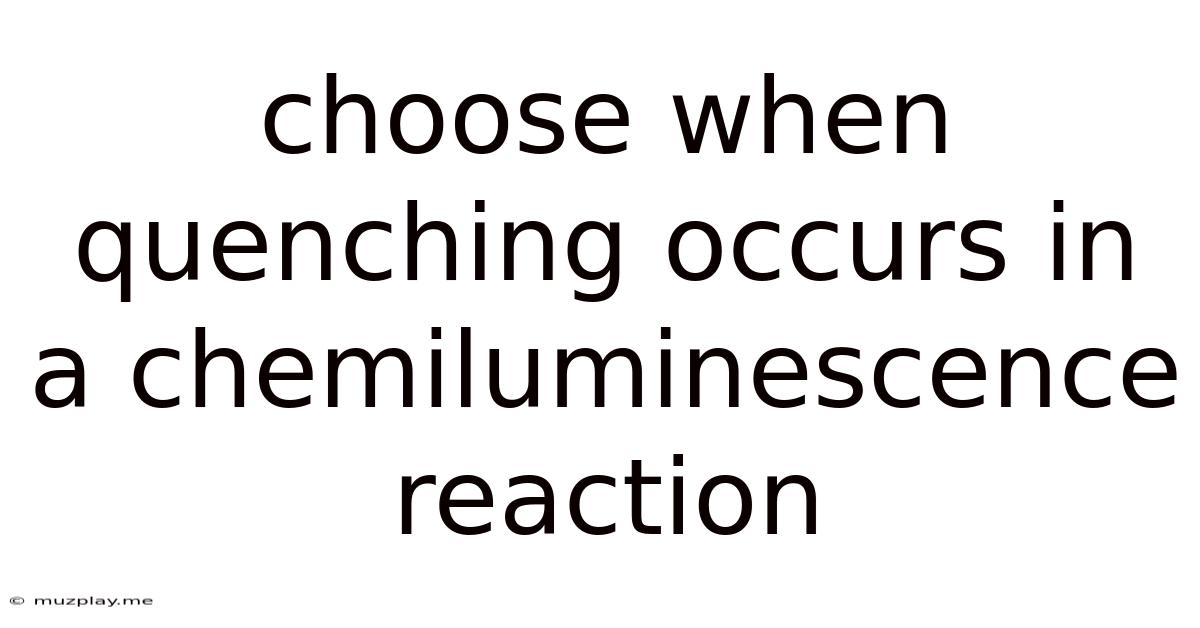
Table of Contents
Choosing the Quenching Moment in Chemiluminescence Reactions: A Comprehensive Guide
Chemiluminescence, the emission of light as a result of a chemical reaction, offers a fascinating window into the world of reactive chemistry and has found widespread applications in various fields, from medical diagnostics to environmental monitoring. A critical aspect of controlling and optimizing chemiluminescence reactions lies in understanding and manipulating the quenching process. Quenching, the reduction in light emission intensity, can significantly impact the overall performance and utility of a chemiluminescent system. This article delves into the complexities of choosing the optimal time for quenching in a chemiluminescence reaction, exploring the factors that influence this crucial decision and providing a comprehensive guide for researchers and practitioners.
Understanding Chemiluminescence and Quenching Mechanisms
Before discussing the timing of quenching, it's essential to grasp the fundamental principles governing chemiluminescence. In a typical chemiluminescent reaction, an excited electronic state is formed during the reaction pathway. This excited state is inherently unstable and rapidly decays to a lower energy state, releasing the excess energy as photons—light. The intensity and duration of this light emission are determined by the kinetics of the reaction and the efficiency of the excited state formation.
Quenching, however, interferes with this process. Quenching agents or processes interrupt the pathway leading to the formation of the excited state or accelerate the non-radiative decay of the excited state to the ground state, thus reducing the light output. Several mechanisms contribute to quenching:
1. Collisional Quenching:
This is perhaps the most common quenching mechanism, where excited state molecules collide with other molecules (quenchers) and transfer their energy non-radiatively. The efficiency of this process depends on the collision frequency and the nature of the quencher. Common quenchers include oxygen, water, and various impurities.
2. Energy Transfer Quenching:
In this mechanism, the excited state transfers its energy to another molecule, leaving the original molecule in its ground state. The acceptor molecule may then fluoresce or phosphoresce, but this is typically at a different wavelength than the original chemiluminescent reaction.
3. Chemical Quenching:
This involves a chemical reaction between the excited state and a quencher molecule, leading to the formation of a new ground state product without light emission.
4. Self-Quenching:
This arises from interactions between the excited state molecules themselves, where collision or energy transfer between excited molecules leads to quenching. This becomes increasingly significant at higher concentrations of the chemiluminescent species.
Factors Influencing the Optimal Quenching Time
The decision of when to quench a chemiluminescence reaction is not arbitrary. It depends on several interconnected factors:
1. Desired Signal-to-Noise Ratio:
The primary goal in many chemiluminescence assays is to achieve a high signal-to-noise ratio (SNR). Early quenching might lead to a weaker signal, while late quenching could result in increased background noise due to the decay of the chemiluminescence and the accumulation of interfering products. The optimal quenching time maximizes the difference between the chemiluminescence signal and the background noise, ensuring accurate and reliable measurements.
2. Reaction Kinetics:
The rate and kinetics of the chemiluminescence reaction dictates the optimal quenching time. Fast reactions might reach their peak intensity quickly, requiring prompt quenching to avoid significant decay. Conversely, slower reactions may benefit from extended periods before quenching to achieve a stronger signal. Understanding the reaction mechanism and kinetics through meticulous experimentation is essential for determining the ideal quenching time.
3. Quenching Efficiency:
The effectiveness of the chosen quenching method significantly influences the decision. A highly efficient quenching method can quickly suppress light emission, allowing for precise control over the signal. However, if the quenching process itself is slow or incomplete, a longer quenching time may be necessary to achieve the desired reduction in light emission.
4. Instrumentation and Detection:
The type of detector used impacts the quenching strategy. Fast detectors, for instance, may allow for shorter integration times, enabling precise measurement even with fast reactions and short-lived chemiluminescence. On the other hand, slow or less sensitive detectors may benefit from a longer observation period before quenching to integrate more light and improve signal quality.
5. Nature of the Chemiluminescence System:
The specific chemiluminescent system employed significantly influences the optimal quenching time. Different chemiluminescent reactions have distinct kinetics and sensitivities to quenching agents. Some reactions might exhibit a rapid initial burst of light followed by a slow decay, requiring a different quenching strategy compared to reactions with a slower and more gradual light emission.
6. Application-Specific Requirements:
The specific application dictates the desired properties of the chemiluminescence signal. For instance, in medical diagnostic assays, a strong and consistent signal is crucial for accurate results, while in some environmental monitoring applications, the total light emission integrated over a period might be more relevant. These application-specific requirements guide the choice of the quenching time.
Strategies for Determining Optimal Quenching Time
Determining the optimal quenching time requires a systematic approach incorporating both theoretical and experimental considerations. Here are some practical strategies:
1. Kinetic Studies:
Conducting detailed kinetic studies of the chemiluminescence reaction helps determine the rate and nature of the light emission. By monitoring the light intensity as a function of time, the peak emission time and the subsequent decay profile can be established, providing crucial insights into the ideal quenching window.
2. Optimization Experiments:
A series of experiments varying the quenching time, while keeping other parameters constant, is critical. Measure the light intensity at different quenching times and quantify the signal-to-noise ratio. This iterative process helps identify the quenching time that maximizes the SNR and minimizes errors.
3. Statistical Analysis:
Statistical analysis of the data collected from optimization experiments helps identify the optimal quenching time with confidence. Techniques like regression analysis can be used to fit the data to a suitable model, identifying the point of maximum signal or optimal SNR.
4. Simulation and Modeling:
Advanced simulations and modelling approaches can be employed to predict the behavior of the chemiluminescence reaction under various quenching conditions. These methods, often based on complex chemical kinetic models, can help narrow down the range of potential quenching times before performing experimental tests.
Examples of Chemiluminescence Reactions and Quenching Considerations
Numerous chemiluminescence reactions find applications in various fields. Let's consider a few examples and explore the specific quenching considerations:
1. Luminol Chemiluminescence:
Luminol, a widely used chemiluminescence reagent, reacts with an oxidant (typically hydrogen peroxide) in an alkaline medium to produce a bright blue light. The reaction is relatively fast and exhibits a rapid initial burst of light, followed by a gradual decay. Quenching is often achieved by adding a strong acid or a reducing agent to neutralize the reaction mixture and terminate light emission. The optimal quenching time in this system needs to be carefully determined to capture the maximum light intensity without significant signal loss or noise increase.
2. Acridinium Ester Chemiluminescence:
Acridinium esters are another class of important chemiluminescent reagents widely used in immunoassays. Their chemiluminescence reactions are characterized by a relatively slow decay rate. The optimal quenching time might be longer compared to Luminol, allowing for more significant light integration and improved sensitivity. However, excessively long delays could lead to increased background noise. Careful optimization is essential to balance these competing factors.
3. Firefly Luciferase Chemiluminescence:
Firefly luciferase catalyzes the oxidation of luciferin, a substrate, producing light. This bioluminescent reaction is characterized by a slower decay, which presents unique challenges to optimization. Quenching is usually achieved through the depletion of luciferin substrate or the addition of a strong inhibitor. The optimal quenching time will depend on factors like substrate concentration, enzyme activity, and desired assay duration.
Conclusion
Choosing the optimal quenching time in chemiluminescence reactions is a critical step in optimizing the performance of these systems. It's a multifaceted process influenced by the kinetics of the reaction, the efficiency of the quenching method, the sensitivity of the detection system, and application-specific needs. A systematic approach incorporating kinetic studies, optimization experiments, statistical analysis, and modelling can greatly facilitate this decision, leading to improved accuracy, sensitivity, and reliability in diverse chemiluminescence applications. Careful consideration of all the factors described in this article ensures effective control of the chemiluminescence process and unlocks its full potential in various scientific and technological advancements.
Latest Posts
Latest Posts
-
Which Formulas Represent Ionic Compounds And Which Represent Covalent Compounds
May 10, 2025
-
When A Glacier Moves Rocks And Sediments
May 10, 2025
-
Which Elements Do Carbohydrates Contain And In What Ratio
May 10, 2025
-
Which Is An Example Of A Colloid
May 10, 2025
-
Difference Between Delta G And Delta G Degree
May 10, 2025
Related Post
Thank you for visiting our website which covers about Choose When Quenching Occurs In A Chemiluminescence Reaction . We hope the information provided has been useful to you. Feel free to contact us if you have any questions or need further assistance. See you next time and don't miss to bookmark.