Chromosomes Line Up Along The Equator Not In Homologous Pairs
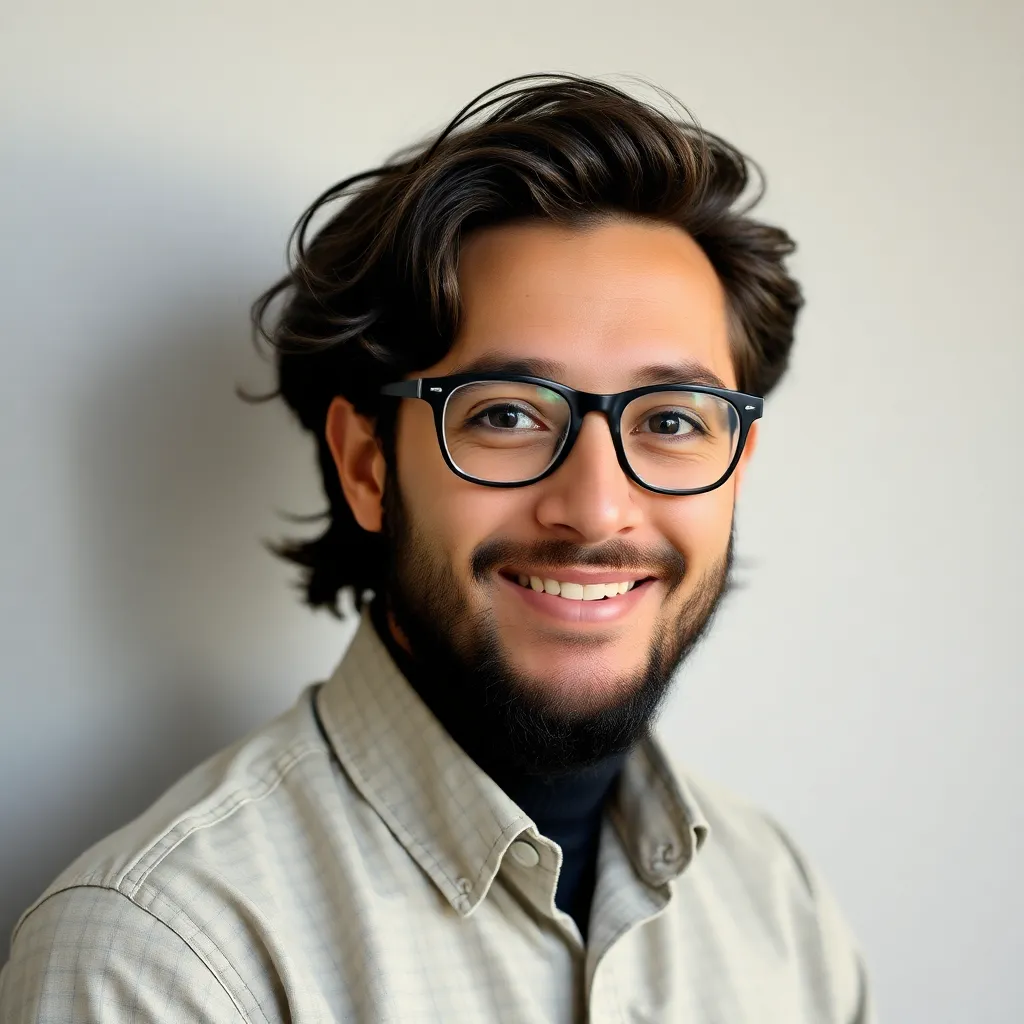
Muz Play
May 09, 2025 · 5 min read
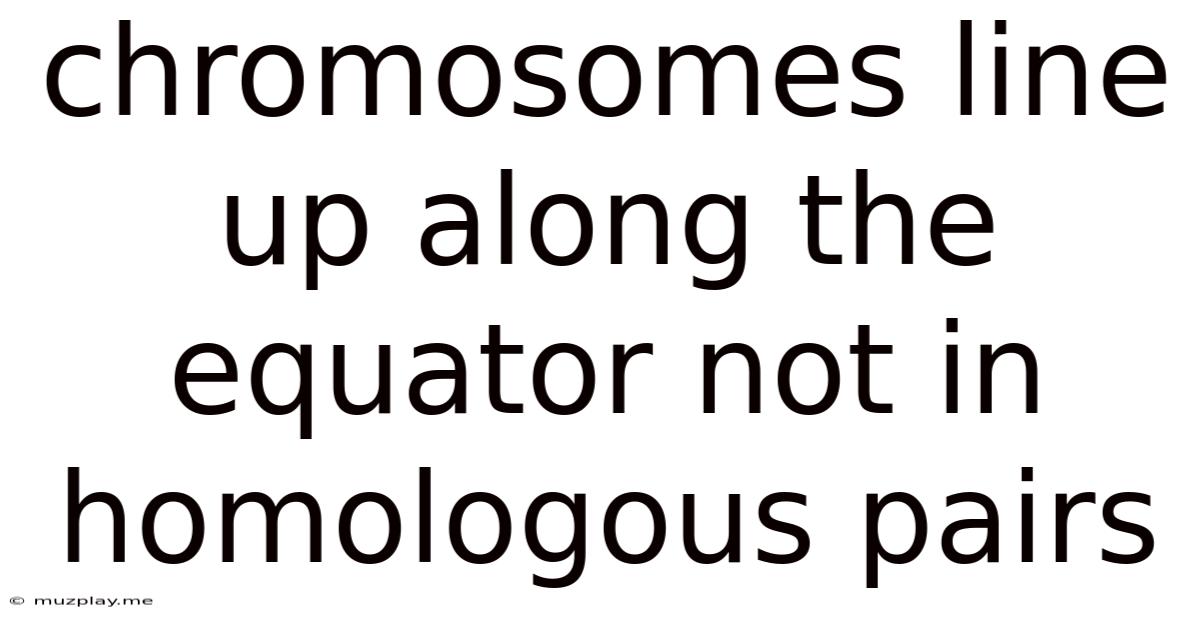
Table of Contents
Chromosomes Line Up Along the Equator: A Deeper Dive into Meiosis I and II
The meticulous dance of chromosomes during cell division is a cornerstone of life itself. While the familiar image depicts homologous chromosomes pairing up during meiosis I, the reality is more nuanced. This article delves into the intricate process of chromosome alignment along the metaphase plate (equator) during both meiosis I and meiosis II, highlighting the crucial differences and the implications for genetic diversity. We'll explore the mechanisms driving this precise arrangement and the consequences of errors in this critical stage.
Meiosis I: A Symphony of Homologous Pair Alignment (and Separation)
Meiosis I is the reductional division, halving the chromosome number. It's characterized by the pairing of homologous chromosomes—one inherited from each parent—to form bivalents. This pairing isn't a random event; it's a precisely orchestrated process involving several key steps:
1. Leptotene: Chromosomes Condense
The prophase I stage begins with leptotene, where chromosomes start to condense, becoming visible under a microscope. At this point, they are not yet paired.
2. Zygotene: Homologous Chromosomes Find Their Partners
In zygotene, homologous chromosomes begin to recognize and pair up, a process called synapsis. The synaptonemal complex, a protein structure, forms between the homologous chromosomes, holding them together tightly.
3. Pachytene: Crossing Over Creates Genetic Diversity
Pachytene is where crossing over occurs. Non-sister chromatids from homologous chromosomes exchange genetic material, creating recombinant chromosomes. This recombination shuffles alleles, contributing significantly to genetic diversity within a population.
4. Diplotene: Chiasmata Mark the Sites of Crossing Over
As the synaptonemal complex disassembles in diplotene, the points of crossing over—called chiasmata—become visible as X-shaped structures. These chiasmata hold the homologous chromosomes together until metaphase I.
5. Diakinesis: Final Condensation Before Alignment
In diakinesis, the chromosomes further condense, and the nuclear envelope breaks down, preparing for the next stage. The chiasmata remain visible, ensuring that homologous chromosomes are connected.
6. Metaphase I: Homologous Pairs Align at the Equator
This is the crucial stage where homologous chromosome pairs align at the metaphase plate, not individual chromosomes. The orientation of each homologous pair is random, a phenomenon called independent assortment. This randomness further contributes to genetic diversity. Microtubules from opposite poles of the cell attach to the kinetochores of each homologous chromosome in the pair, preparing for their separation.
7. Anaphase I: Homologous Chromosomes Separate
In anaphase I, the homologous chromosomes are pulled apart and move to opposite poles of the cell. Crucially, sister chromatids remain attached at the centromere; it's the homologous pairs that separate.
8. Telophase I and Cytokinesis: Two Haploid Cells are Formed
Telophase I sees the formation of two daughter cells, each containing a haploid set of chromosomes. Cytokinesis, the division of the cytoplasm, completes the process. Importantly, each daughter cell contains a unique combination of chromosomes due to independent assortment and crossing over.
Meiosis II: Sister Chromatids Part Ways
Meiosis II is an equational division; it's similar to mitosis. The key difference lies in the starting point: we begin with haploid cells.
1. Prophase II: Chromosomes Condense Again
The chromosomes in each haploid cell condense once more.
2. Metaphase II: Individual Chromosomes Align at the Equator
This is where the crucial difference from meiosis I becomes apparent. In metaphase II, individual chromosomes, not homologous pairs, align at the metaphase plate. The kinetochores of sister chromatids are attached to microtubules from opposite poles.
3. Anaphase II: Sister Chromatids Separate
In anaphase II, the sister chromatids finally separate, and each chromatid (now considered a chromosome) moves to opposite poles.
4. Telophase II and Cytokinesis: Four Haploid Gametes Result
Telophase II and cytokinesis produce four haploid daughter cells, each with a unique combination of chromosomes. These are the gametes (sperm or egg cells) that will participate in fertilization.
The Significance of Precise Chromosome Alignment
The precise alignment of chromosomes during both meiosis I and II is essential for accurate chromosome segregation. Errors in this process can lead to:
- Aneuploidy: An abnormal number of chromosomes in a cell. This can result in developmental disorders, such as Down syndrome (trisomy 21).
- Non-disjunction: Failure of chromosomes to separate correctly during anaphase I or II. This is a common cause of aneuploidy.
- Chromosomal abnormalities: Structural changes in chromosomes, such as deletions, duplications, inversions, and translocations. These can also lead to developmental problems or infertility.
The mechanisms ensuring proper alignment are complex and involve a multitude of proteins, including:
- Cohesins: Proteins that hold sister chromatids together.
- Kinetochores: Protein structures on chromosomes where microtubules attach.
- Microtubules: Protein filaments that form the spindle apparatus, responsible for chromosome movement.
- Motor proteins: Proteins that generate the forces required for chromosome movement.
Errors and Their Consequences: A Closer Look
While the cell has robust mechanisms to prevent errors in chromosome alignment, they can still occur. Factors that increase the risk of errors include:
- Age: The risk of non-disjunction increases with maternal age, particularly for meiosis I errors.
- Genetic predisposition: Certain genetic mutations can increase the risk of chromosome segregation errors.
- Environmental factors: Exposure to certain chemicals or radiation can damage chromosomes and increase the risk of errors.
The consequences of these errors can be severe, ranging from spontaneous miscarriage to birth defects and developmental disabilities. Understanding the molecular mechanisms underlying chromosome alignment and segregation is therefore crucial for developing strategies to prevent these errors and improve reproductive health outcomes.
Conclusion: The Intricate Choreography of Meiosis
The alignment of chromosomes along the metaphase plate during meiosis I and II is a highly regulated and intricate process. While the visual representation often simplifies the process, showing homologous pairs neatly aligned in meiosis I and individual chromosomes in meiosis II, the underlying mechanisms are vastly complex and essential for generating the genetic diversity necessary for evolution and survival. Errors in this delicate dance have profound consequences, emphasizing the importance of research into this fundamental biological process. Future studies will undoubtedly uncover further details about the intricate molecular machinery that ensures the accurate segregation of chromosomes, paving the way for better understanding of reproductive health and genetic disorders.
Latest Posts
Latest Posts
-
How To Find A Function From An Equation
May 09, 2025
-
What Are The Subunits Of Nucleic Acids
May 09, 2025
-
How To Find The Max Of A Parabola
May 09, 2025
-
What Do Sodium And Chlorine Make When Combined
May 09, 2025
-
Identify Two Factors That Can Contribute To Violence
May 09, 2025
Related Post
Thank you for visiting our website which covers about Chromosomes Line Up Along The Equator Not In Homologous Pairs . We hope the information provided has been useful to you. Feel free to contact us if you have any questions or need further assistance. See you next time and don't miss to bookmark.