Classify Each Of The Following Organic Reactions
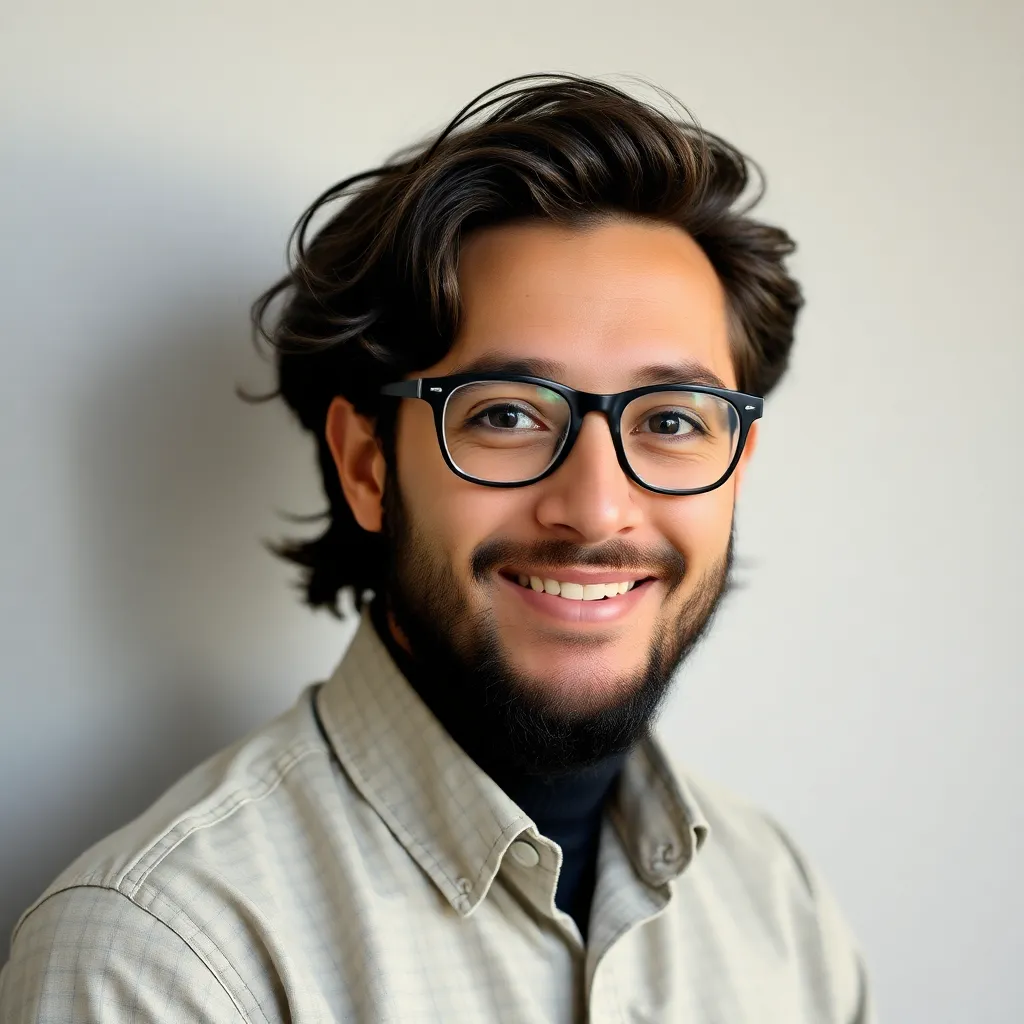
Muz Play
Apr 27, 2025 · 6 min read

Table of Contents
Classify Each of the Following Organic Reactions: A Comprehensive Guide
Organic chemistry, the study of carbon-containing compounds, is built upon a foundation of countless reactions. Understanding the classification of these reactions is crucial for predicting their outcomes, designing synthetic pathways, and generally mastering the subject. This article will delve into various organic reaction classifications, providing detailed explanations and examples. We'll cover the major reaction types – addition, elimination, substitution, and rearrangement – and explore their nuances with specific examples.
1. Addition Reactions
Addition reactions are characterized by the combination of two or more molecules to form a larger one. Typically, this occurs with unsaturated compounds containing double or triple bonds (alkenes, alkynes). The pi bonds break, and new sigma bonds are formed, increasing the saturation of the molecule.
1.1 Electrophilic Addition
This is a common addition reaction where an electrophile, an electron-deficient species, attacks the electron-rich pi bond of an alkene or alkyne. The electrophile adds to one carbon atom, forming a carbocation intermediate. This intermediate is then attacked by a nucleophile, an electron-rich species.
Example: The addition of hydrogen bromide (HBr) to propene:
The HBr molecule is polarized, with hydrogen carrying a slightly positive charge and bromine a slightly negative charge. The electrophilic hydrogen attacks the double bond, forming a carbocation intermediate. The nucleophilic bromide ion then attacks the carbocation, resulting in 2-bromopropane. This follows Markovnikov's rule, which states that the hydrogen atom adds to the carbon atom with more hydrogen atoms already attached.
1.2 Nucleophilic Addition
In nucleophilic addition, a nucleophile, an electron-rich species, attacks an electron-deficient carbon atom. This is commonly seen in carbonyl compounds (aldehydes, ketones, carboxylic acids, esters). The nucleophile adds to the carbonyl carbon, forming a new bond and often resulting in a tetrahedral intermediate.
Example: The addition of a Grignard reagent (RMgX) to a ketone:
The Grignard reagent acts as a nucleophile, attacking the carbonyl carbon of the ketone. This forms a tetrahedral intermediate, which subsequently undergoes protonation to yield a tertiary alcohol.
1.3 Free Radical Addition
Free radical addition involves the addition of a free radical, a species with an unpaired electron, to an alkene or alkyne. This often involves initiation, propagation, and termination steps.
Example: The addition of HBr to propene in the presence of peroxides:
The peroxide initiates the reaction by forming bromine radicals. These radicals then add to the double bond, leading to a different regioselectivity compared to electrophilic addition (Anti-Markovnikov's rule).
2. Elimination Reactions
Elimination reactions involve the removal of atoms or groups from a molecule to form a double or triple bond. They are the reverse of addition reactions. Two common types are E1 and E2 eliminations.
2.1 E1 Elimination (Unimolecular Elimination)
E1 eliminations are first-order reactions, meaning the rate depends only on the concentration of the substrate. They typically involve a two-step mechanism:
- Formation of a carbocation: A leaving group departs, forming a carbocation intermediate.
- Deprotonation: A base abstracts a proton from a carbon adjacent to the carbocation, resulting in the formation of a double bond.
Example: The dehydration of an alcohol:
Heating an alcohol in the presence of an acid catalyst leads to the formation of an alkene through an E1 elimination mechanism. The acid protonates the hydroxyl group, making it a better leaving group. The subsequent departure of water forms a carbocation, which is deprotonated by another molecule of the alcohol or a conjugate base.
2.2 E2 Elimination (Bimolecular Elimination)
E2 eliminations are second-order reactions, meaning the rate depends on the concentration of both the substrate and the base. They occur in a single step, where the base abstracts a proton and the leaving group departs simultaneously, resulting in the formation of a double bond.
Example: The dehydrohalogenation of an alkyl halide:
Treating an alkyl halide with a strong base, like potassium tert-butoxide, leads to an E2 elimination, forming an alkene. The strong base abstracts a proton anti-periplanar to the leaving group, facilitating a concerted elimination.
3. Substitution Reactions
Substitution reactions involve the replacement of one atom or group with another. They are classified into several types based on the mechanism and nature of the reactants.
3.1 SN1 Reactions (Unimolecular Nucleophilic Substitution)
SN1 reactions are first-order reactions, meaning the rate depends only on the concentration of the substrate. They proceed through a two-step mechanism:
- Formation of a carbocation: The leaving group departs, forming a carbocation intermediate.
- Nucleophilic attack: A nucleophile attacks the carbocation, forming a new bond.
Example: The solvolysis of tert-butyl bromide in water:
In this reaction, the bromide ion leaves, forming a tertiary carbocation. Water then attacks the carbocation, forming a tert-butyl alcohol. SN1 reactions favor tertiary substrates due to the greater stability of tertiary carbocations.
3.2 SN2 Reactions (Bimolecular Nucleophilic Substitution)
SN2 reactions are second-order reactions, meaning the rate depends on the concentration of both the substrate and the nucleophile. They occur in a single step, where the nucleophile attacks the substrate from the backside, simultaneously displacing the leaving group. This leads to inversion of configuration at the stereocenter.
Example: The reaction of bromomethane with hydroxide ion:
The hydroxide ion attacks the carbon atom bearing the bromine, displacing the bromide ion and forming methanol. SN2 reactions favor primary substrates due to steric hindrance.
3.3 Electrophilic Aromatic Substitution
Electrophilic aromatic substitution involves the replacement of a hydrogen atom on an aromatic ring with an electrophile. This occurs through a multi-step mechanism involving the formation of a resonance-stabilized carbocation intermediate.
Example: Nitration of benzene:
Benzene reacts with a nitronium ion (NO2+), generated from nitric acid and sulfuric acid, to form nitrobenzene. The nitronium ion attacks the benzene ring, forming a resonance-stabilized carbocation, which is then deprotonated to regain aromaticity.
4. Rearrangement Reactions
Rearrangement reactions involve the intramolecular reorganization of atoms within a molecule. These rearrangements often aim to increase the stability of the molecule. Several types of rearrangements exist, including:
4.1 Claisen Rearrangement
The Claisen rearrangement involves the [3,3]-sigmatropic rearrangement of an allyl vinyl ether to form a γ,δ-unsaturated carbonyl compound. This reaction is thermally driven and proceeds through a concerted mechanism.
4.2 Cope Rearrangement
The Cope rearrangement involves the [3,3]-sigmatropic rearrangement of a 1,5-diene to form an isomeric 1,5-diene. This reaction is also thermally driven and concerted.
4.3 Wagner-Meerwein Rearrangement
The Wagner-Meerwein rearrangement involves the migration of an alkyl or aryl group from one carbon atom to an adjacent carbon atom in a carbocation intermediate. This often occurs during solvolysis or other reactions that form carbocations.
Conclusion
This article provides a broad overview of the major classifications of organic reactions. Understanding these classifications, along with the mechanisms involved, is fundamental to success in organic chemistry. Further exploration of specific reactions within each category will undoubtedly deepen your understanding and enable you to predict and design organic syntheses effectively. Remember, practice is key – work through various examples and problems to solidify your grasp of these concepts. By mastering these classifications, you'll be well-equipped to tackle the complexities of organic chemistry and excel in your studies.
Latest Posts
Latest Posts
-
Is Table Salt A Mixture Or A Compound
Apr 28, 2025
-
Find Each Measure M1 M2 M3
Apr 28, 2025
-
What Two Monosaccharides Make Up Lactose
Apr 28, 2025
-
How Do You Create A Common Size Balance Sheet
Apr 28, 2025
-
Counting Valence Electrons In A Molecule Or Polyatomic Ion
Apr 28, 2025
Related Post
Thank you for visiting our website which covers about Classify Each Of The Following Organic Reactions . We hope the information provided has been useful to you. Feel free to contact us if you have any questions or need further assistance. See you next time and don't miss to bookmark.