Compare And Contrast Primary And Secondary Active Transport
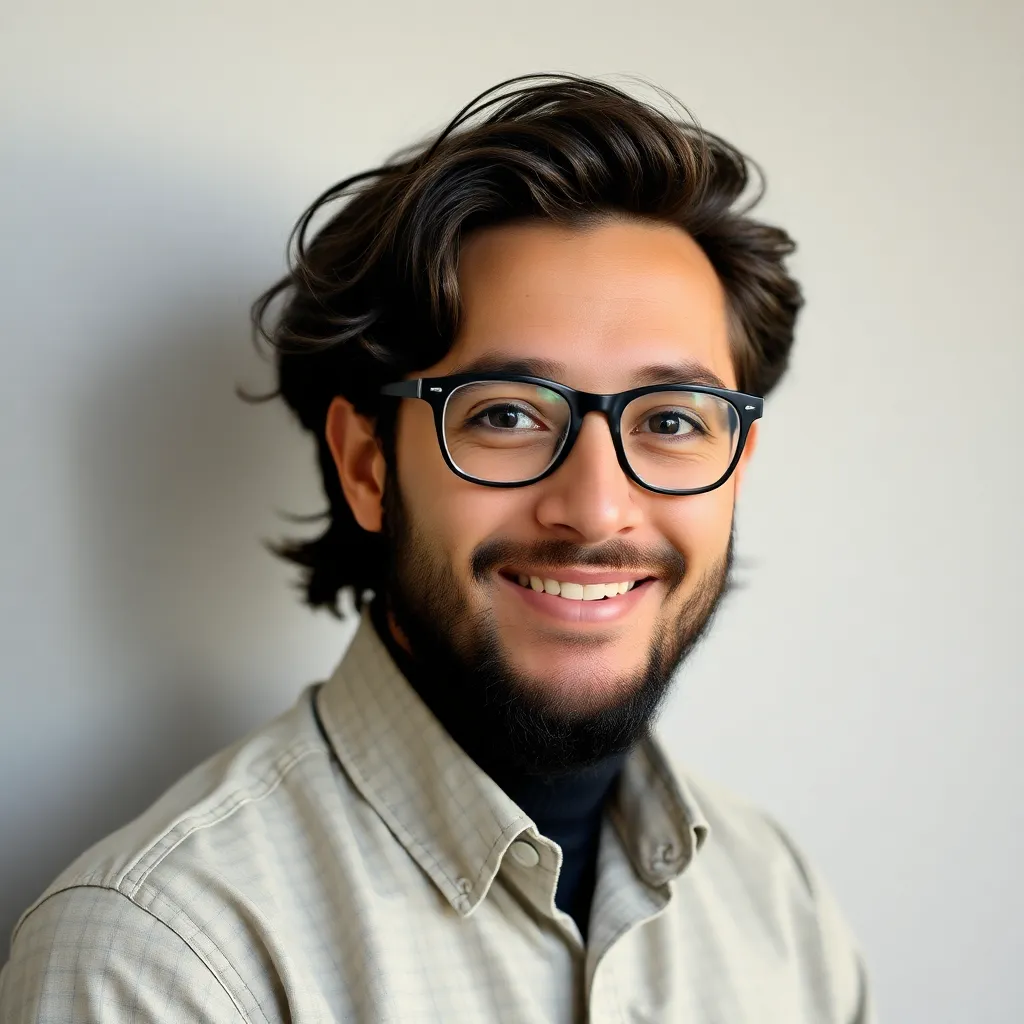
Muz Play
Apr 20, 2025 · 6 min read

Table of Contents
Comparing and Contrasting Primary and Secondary Active Transport: A Deep Dive into Cellular Mechanisms
Active transport, a vital process in cellular biology, allows cells to move molecules across their membranes against their concentration gradients. This energy-intensive process is crucial for maintaining cellular homeostasis and enabling various physiological functions. Two primary categories define active transport: primary and secondary active transport. While both move molecules uphill, their mechanisms and energy sources differ significantly. This detailed exploration will meticulously compare and contrast these two fundamental processes.
Understanding the Fundamentals of Active Transport
Before diving into the specifics of primary and secondary active transport, it's essential to grasp the basic principles of active transport itself. Active transport, unlike passive transport (diffusion, osmosis, facilitated diffusion), requires energy to function. This is because it moves substances against their concentration gradients – from an area of low concentration to an area of high concentration. This "uphill" movement necessitates an input of energy to overcome the natural tendency for molecules to move down their concentration gradients.
The Role of Membrane Proteins
Central to active transport are membrane proteins, specifically transmembrane proteins. These proteins act as carriers or pumps, binding to the transported molecule and facilitating its movement across the membrane. Their specificity ensures that only certain molecules are transported, allowing for selective control over cellular uptake and efflux.
Energy Sources for Active Transport
The critical distinction between primary and secondary active transport lies in their energy sources. Primary active transport directly utilizes energy from the hydrolysis of ATP (adenosine triphosphate), the cell's primary energy currency. Secondary active transport, however, harnesses the energy stored in an electrochemical gradient established by primary active transport.
Primary Active Transport: Direct Energy Utilization
Primary active transport directly couples the movement of a molecule against its concentration gradient to the hydrolysis of ATP. The energy released during ATP hydrolysis provides the force necessary to drive the transport process. This direct linkage is the defining characteristic of primary active transport.
The Sodium-Potassium Pump (Na+/K+-ATPase): A Prime Example
The most well-known and extensively studied example of primary active transport is the sodium-potassium pump (Na+/K+-ATPase). This ubiquitous protein pump plays a critical role in maintaining the electrochemical gradients of sodium (Na+) and potassium (K+) ions across the cell membrane. The pump actively transports three Na+ ions out of the cell and two K+ ions into the cell for every molecule of ATP hydrolyzed.
Mechanism of the Na+/K+-ATPase:
- Binding of Na+: Three intracellular Na+ ions bind to specific sites on the pump.
- ATP Hydrolysis: A molecule of ATP binds to the pump and is hydrolyzed, releasing energy. This energy causes a conformational change in the pump.
- Na+ Translocation: The conformational change moves the three Na+ ions across the membrane to the extracellular space.
- Binding of K+: Two extracellular K+ ions bind to the pump.
- Phosphate Release: The phosphate group released during ATP hydrolysis is released, causing another conformational change.
- K+ Translocation: The conformational change moves the two K+ ions across the membrane to the intracellular space.
- Cycle Repetition: The pump returns to its original conformation, ready to repeat the cycle.
Other Examples of Primary Active Transport:
Besides the Na+/K+-ATPase, other examples of primary active transport include:
- Ca2+-ATPase: Pumps calcium ions (Ca2+) out of the cell or into intracellular stores, maintaining low cytosolic Ca2+ concentrations. Essential for muscle contraction and signal transduction.
- H+/K+-ATPase: Located in parietal cells of the stomach, this pump secretes H+ ions into the stomach lumen, contributing to gastric acidification. Crucial for digestion.
- ABC Transporters (ATP-Binding Cassette Transporters): A large superfamily of transporters that utilize ATP hydrolysis to transport a wide range of molecules, including drugs, lipids, and peptides. Involved in drug resistance and various metabolic pathways.
Secondary Active Transport: Indirect Energy Utilization
Secondary active transport utilizes the energy stored in an electrochemical gradient created by primary active transport, rather than directly hydrolyzing ATP. This gradient, typically the Na+ gradient established by the Na+/K+-ATPase, provides the driving force for the movement of other molecules.
The Power of Electrochemical Gradients
The Na+ gradient established by the Na+/K+-ATPase has a higher concentration of Na+ outside the cell and a higher concentration of K+ inside the cell. This creates both a concentration gradient and an electrical gradient (membrane potential). The combined effect is an electrochemical gradient, which stores potential energy.
Symporters and Antiporters: Two Types of Secondary Active Transport
Secondary active transport proteins are broadly categorized into two groups based on the direction of movement of the transported molecules relative to the ion used to generate the electrochemical gradient:
- Symporters (cotransporters): These transporters move two molecules in the same direction across the membrane. One molecule moves down its electrochemical gradient (typically Na+), providing the energy to move the other molecule against its gradient.
- Antiporters (exchangers): These transporters move two molecules in opposite directions across the membrane. One molecule moves down its electrochemical gradient, providing the energy to move the other molecule against its gradient.
Examples of Secondary Active Transport:
- Sodium-glucose cotransporter (SGLT1): A symporter located in the intestinal epithelium. The movement of Na+ down its gradient drives the uptake of glucose against its gradient, facilitating glucose absorption.
- Sodium-calcium exchanger (NCX): An antiporter that removes Ca2+ from the cell by utilizing the inward movement of Na+. Crucial in maintaining low intracellular Ca2+ levels in various cell types.
- Sodium-proton exchanger (NHE): An antiporter that removes H+ ions from the cell in exchange for Na+ entry. Important in regulating intracellular pH.
A Comparative Table: Primary vs. Secondary Active Transport
Feature | Primary Active Transport | Secondary Active Transport |
---|---|---|
Energy Source | Direct ATP hydrolysis | Electrochemical gradient (indirect) |
ATP Involvement | Direct and required | Indirect, ATP used to create the gradient |
Transport Proteins | ATPases (e.g., Na+/K+-ATPase) | Symporters and antiporters |
Gradient Creation | Creates electrochemical gradients | Utilizes pre-existing electrochemical gradients |
Examples | Na+/K+-ATPase, Ca2+-ATPase, H+/K+-ATPase | SGLT1, NCX, NHE |
Efficiency | Less efficient due to direct ATP cost | More efficient, using pre-existing energy |
Conclusion: Interdependence and Cellular Significance
Primary and secondary active transport are intricately linked processes essential for maintaining cellular function. Primary active transport creates the electrochemical gradients that drive secondary active transport. This collaboration highlights the elegant efficiency of cellular mechanisms. The various types of active transport, each with their specific roles and substrates, underscore the complexity and precision of cellular processes in maintaining life. The intricate dance of these transport systems is paramount to the survival and proper function of all living cells. Further research into these mechanisms promises to unravel deeper insights into cellular processes and disease states. Understanding the nuances of primary and secondary active transport provides a strong foundation for comprehending the broader field of cellular physiology and its implications in human health.
Latest Posts
Latest Posts
-
Nonverbal Communication Is Not Categorized By
Apr 20, 2025
-
Why Do Onions Have No Chloroplasts
Apr 20, 2025
-
Where Are The Respiratory Control Centers Located
Apr 20, 2025
-
The Most Reactive Group Of The Nonmetals Are The
Apr 20, 2025
-
Difference Between Molecular And Chemical Formula
Apr 20, 2025
Related Post
Thank you for visiting our website which covers about Compare And Contrast Primary And Secondary Active Transport . We hope the information provided has been useful to you. Feel free to contact us if you have any questions or need further assistance. See you next time and don't miss to bookmark.