Comparing Chromosome Separation In Bacteria And Eukaryotes
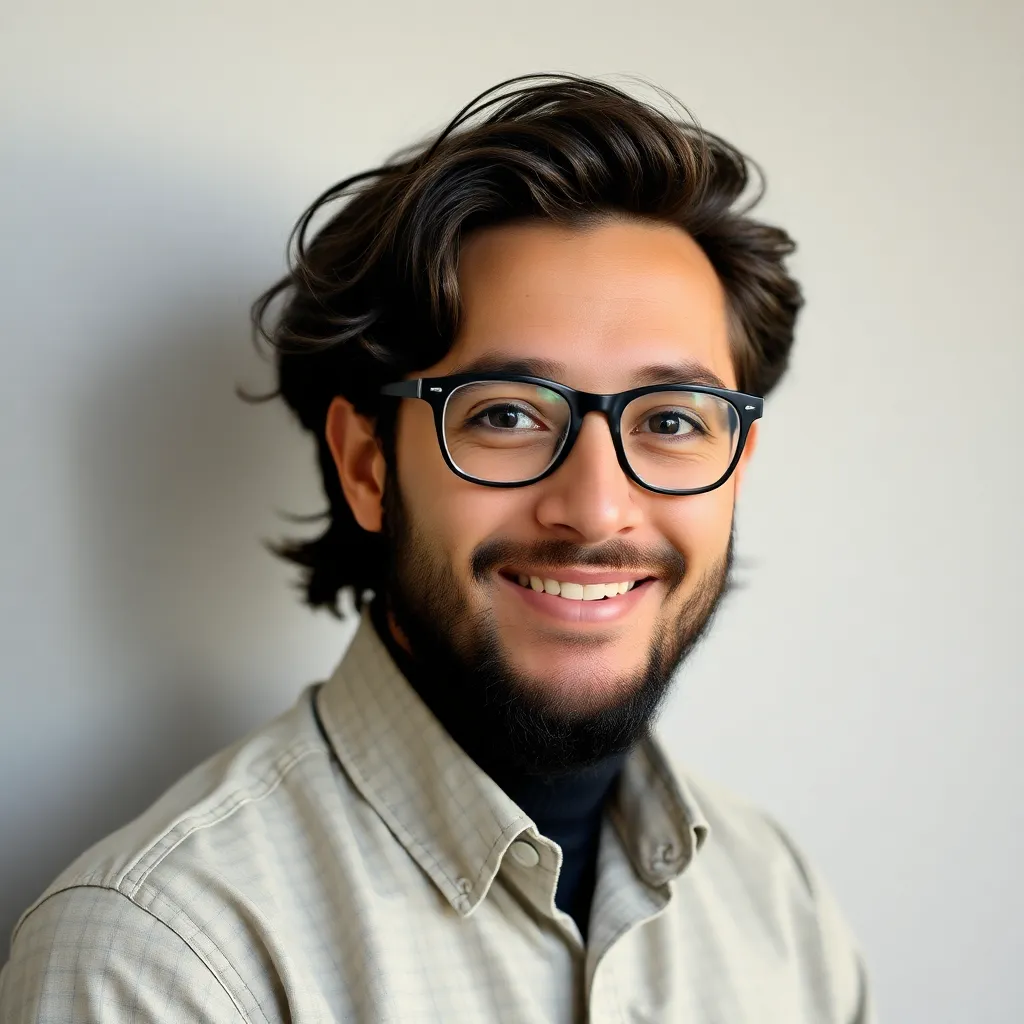
Muz Play
May 11, 2025 · 6 min read
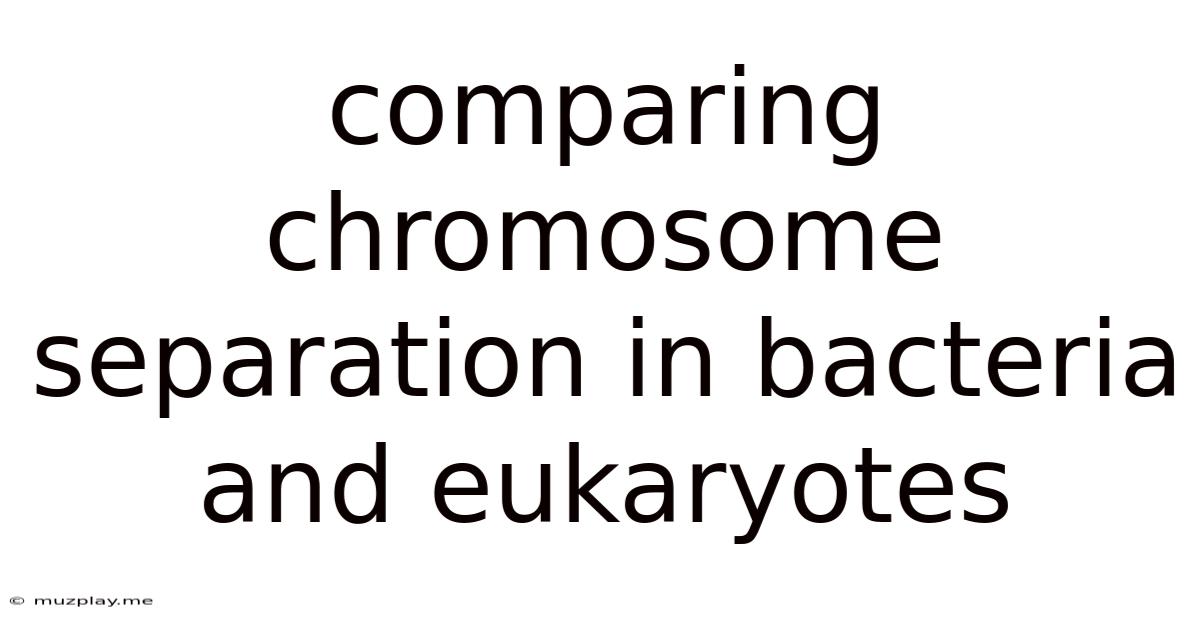
Table of Contents
Comparing Chromosome Separation in Bacteria and Eukaryotes: A Detailed Analysis
Chromosome segregation, the process by which duplicated chromosomes are accurately distributed to daughter cells during cell division, is a fundamental process in all life forms. While the overarching goal is the same – ensuring genetic fidelity – the mechanisms employed by bacteria and eukaryotes differ significantly, reflecting the vast differences in their cellular organization and complexity. This article delves into a detailed comparison of chromosome separation in these two domains of life, exploring the key players, processes, and evolutionary implications.
The Bacterial Chromosome: Simplicity and Efficiency
Bacterial chromosomes are significantly simpler than their eukaryotic counterparts. Typically, bacteria possess a single, circular chromosome located in a region of the cytoplasm called the nucleoid. This chromosome is not enclosed within a membrane-bound nucleus, a defining characteristic of eukaryotic cells. The lack of a nucleus necessitates a streamlined mechanism for chromosome segregation.
Key Players in Bacterial Chromosome Segregation:
-
ParS/ParB System: Many bacteria utilize a sophisticated system involving a centromere-like site called parS on the chromosome and a protein called ParB. ParB binds to parS and plays a crucial role in chromosome dimer resolution and segregation. It helps to organize the chromosome and facilitates its movement towards the cell poles.
-
ParA ATPase: ParA is an ATPase that interacts with ParB and contributes to the directional movement of the chromosomes. It forms filaments and generates force to drive chromosome segregation. The exact mechanism of force generation remains an active area of research, but models suggest it involves dynamic assembly and disassembly of ParA filaments.
-
Topoisomerases: These enzymes are essential for managing the topological challenges associated with DNA replication and segregation. They resolve DNA supercoiling and prevent DNA tangling, ensuring smooth chromosome separation.
-
MreB cytoskeleton: This bacterial homologue of actin plays an important role in chromosome segregation by organizing the nucleoid and influencing the positioning of the segregation machinery. It aids in maintaining cell shape and contributes to the spatial organization required for efficient chromosome partitioning.
-
MukBEF system: Some bacteria utilize the MukBEF system, a distinct system that contributes to chromosome segregation and condensation. This system comprises three proteins: MukB, MukE, and MukF, which interact to organize the bacterial nucleoid and regulate chromosome dynamics.
The Process of Bacterial Chromosome Segregation:
-
Replication Initiation: Chromosome replication begins at a specific origin of replication (oriC). This process generates two identical copies of the chromosome.
-
Chromosome Condensation & Organization: Proteins like MukBEF and MreB contribute to chromosome condensation and organization, preventing tangling and preparing the chromosomes for segregation.
-
Active Segregation: The ParABS system plays a critical role in actively segregating the replicated chromosomes. ParB binds to parS sites, and ParA facilitates their movement towards opposite cell poles.
-
Termination and Cell Division: Chromosome segregation completes prior to cell division. The cell then divides into two daughter cells, each receiving a complete copy of the chromosome.
The Eukaryotic Chromosome: Complexity and Regulation
Eukaryotic chromosomes are significantly more complex than bacterial chromosomes. They are linear, not circular, and are typically present in multiple copies (e.g., humans have 23 pairs). Moreover, they are located within a membrane-bound nucleus, adding another layer of complexity to the segregation process.
Key Players in Eukaryotic Chromosome Segregation:
-
Centromeres: These specialized regions of chromosomes are crucial for chromosome segregation. They serve as attachment points for kinetochores, protein complexes that mediate the interaction between chromosomes and the mitotic spindle.
-
Kinetochores: These complex protein structures assemble on the centromeres and bind to microtubules of the mitotic spindle. They are essential for capturing, aligning, and segregating chromosomes during mitosis and meiosis.
-
Microtubules: These dynamic protein filaments form the mitotic spindle, the apparatus that drives chromosome segregation. They emanate from centrosomes, organizing centers located near the nucleus.
-
Motor Proteins: Various motor proteins, such as kinesins and dyneins, move along microtubules, contributing to chromosome movement and spindle dynamics.
-
Cohesins: These protein complexes hold sister chromatids together from the time of DNA replication until anaphase. They are crucial for ensuring accurate chromosome segregation.
-
Separases: These proteases cleave cohesins, allowing sister chromatids to separate during anaphase. Their activity is tightly regulated to ensure timely chromosome separation.
-
Checkpoints: Eukaryotic cells employ checkpoints to monitor the fidelity of chromosome segregation. These checkpoints halt cell cycle progression if errors are detected, preventing the generation of aneuploid cells with an abnormal number of chromosomes.
The Process of Eukaryotic Chromosome Segregation:
-
DNA Replication: During the S phase of the cell cycle, each chromosome is replicated, generating two identical sister chromatids.
-
Prophase: Chromosomes condense, and the mitotic spindle begins to form. Kinetochores assemble on the centromeres.
-
Metaphase: Chromosomes align at the metaphase plate, an equatorial plane midway between the two spindle poles. Each kinetochore attaches to microtubules from opposite poles, ensuring that sister chromatids will be pulled apart towards opposite poles.
-
Anaphase: Cohesins are cleaved by separases, allowing sister chromatids to separate and move towards opposite poles.
-
Telophase & Cytokinesis: Chromosomes arrive at the poles, decondense, and nuclear envelopes reform. Cytokinesis follows, dividing the cytoplasm and producing two daughter cells, each with a complete set of chromosomes.
A Comparative Overview: Key Differences
Feature | Bacteria | Eukaryotes |
---|---|---|
Chromosome Shape | Circular | Linear |
Chromosome Number | Typically one | Multiple (diploid, etc.) |
Location | Nucleoid (cytoplasm) | Nucleus |
Segregation Mechanism | ParABS system, MreB, MukBEF; mostly active | Mitotic spindle, kinetochores; mostly passive |
Centromeres | Absent (or functionally different) | Present, essential for kinetochore attachment |
Spindle | Absent | Present, composed of microtubules |
Checkpoints | Less elaborate | Extensive checkpoints regulate segregation |
Complexity | Relatively simple | Highly complex |
Evolutionary Implications and Future Research
The differences in chromosome segregation mechanisms between bacteria and eukaryotes reflect their evolutionary trajectories. The simpler bacterial system likely reflects an early evolutionary strategy for chromosome segregation, adapted to the relatively small size and simpler cellular organization of bacterial cells. The evolution of a nucleus and more complex cytoskeleton in eukaryotes necessitated the development of the more sophisticated mitotic spindle apparatus.
Future research will continue to refine our understanding of both bacterial and eukaryotic chromosome segregation. Studies focusing on the precise mechanisms of force generation in bacterial segregation, the intricacies of kinetochore-microtubule interactions in eukaryotes, and the evolutionary relationships between different segregation systems will further illuminate the fundamental principles underlying this crucial process. Understanding these mechanisms is crucial not only for basic biology but also for addressing human diseases linked to chromosome segregation errors, such as cancer. Moreover, uncovering the detailed mechanisms of chromosome segregation in bacteria is increasingly relevant in biotechnology applications, particularly in the field of synthetic biology where precise control over genome manipulation is essential.
The field of chromosome segregation is dynamic, with ongoing research revealing new details and challenging existing models. By continuing to explore the intricacies of this essential process in both bacteria and eukaryotes, we gain a deeper understanding of the fundamental mechanisms that underpin the continuity of life itself. This research holds significant implications for advancing our knowledge of cell biology, evolution, and human health.
Latest Posts
Related Post
Thank you for visiting our website which covers about Comparing Chromosome Separation In Bacteria And Eukaryotes . We hope the information provided has been useful to you. Feel free to contact us if you have any questions or need further assistance. See you next time and don't miss to bookmark.