Complete Oxidative Breakdown Of Glucose Results In
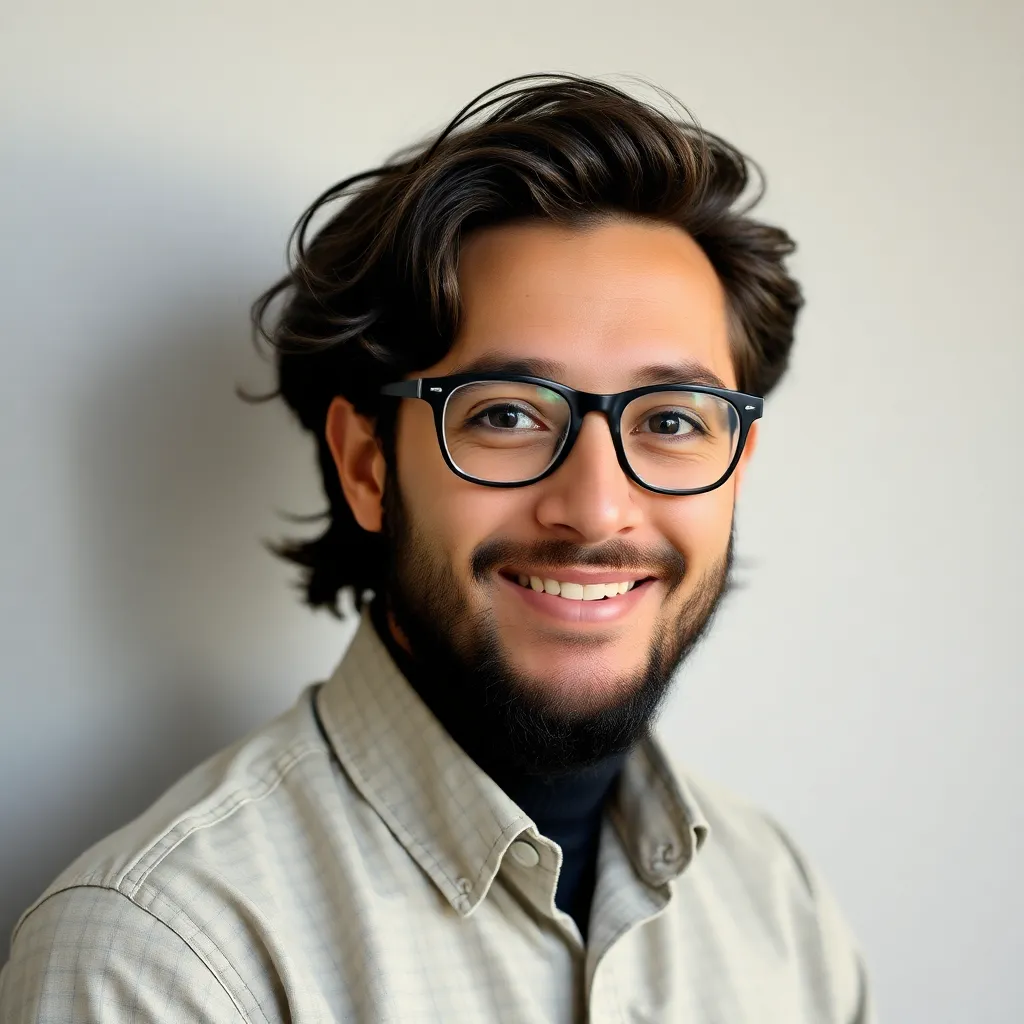
Muz Play
May 10, 2025 · 6 min read
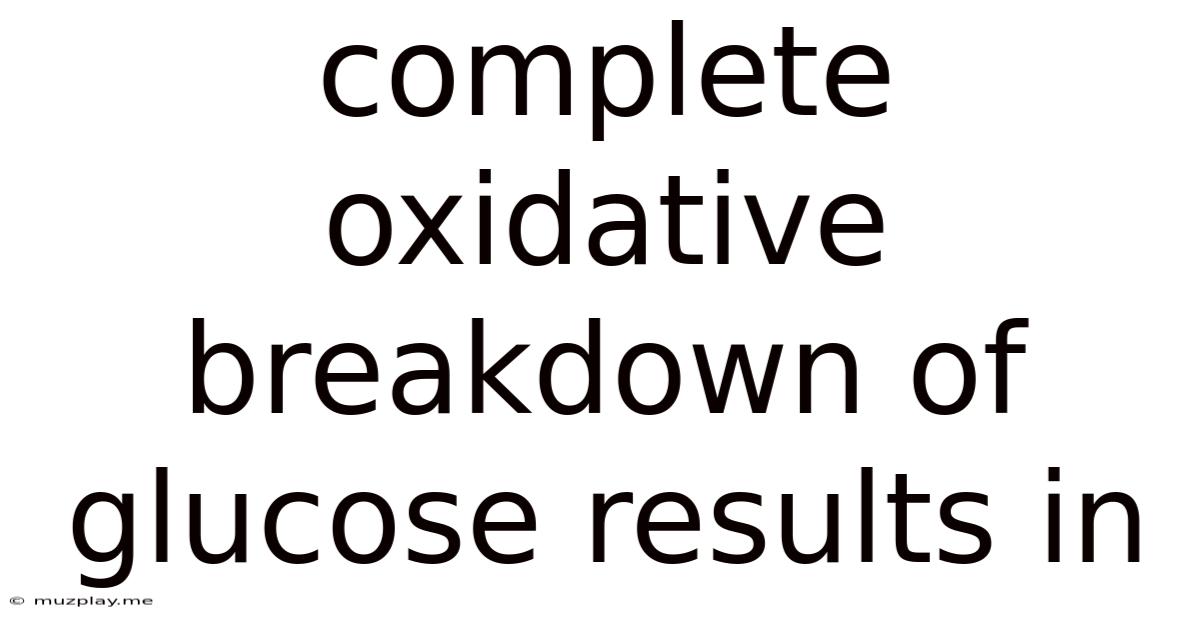
Table of Contents
Complete Oxidative Breakdown of Glucose Results In: A Deep Dive into Cellular Respiration
The complete oxidative breakdown of glucose, a process also known as cellular respiration, is the central energy-generating pathway in most living organisms. It's a marvel of biochemical engineering, meticulously converting the chemical energy stored within glucose into a readily usable form: ATP (adenosine triphosphate). Understanding this intricate process is crucial for grasping the fundamental principles of metabolism and the intricate workings of life itself. This comprehensive article will explore the complete oxidative breakdown of glucose, delving into its stages, key enzymes, regulatory mechanisms, and overall significance.
Stage 1: Glycolysis – The Preparatory Phase
Glycolysis, meaning "sugar splitting," is the initial stage of glucose oxidation, occurring in the cytoplasm of the cell. This anaerobic process doesn't require oxygen and yields a modest amount of ATP. Let's break down the key steps:
Investment Phase: Priming the Pump
The initial steps of glycolysis involve the investment of two ATP molecules per glucose molecule. This seemingly counterintuitive step is essential for activating the glucose molecule and setting the stage for subsequent energy-yielding reactions. Key enzymes involved in this phase include hexokinase and phosphofructokinase, which catalyze crucial phosphorylation reactions.
Payoff Phase: ATP Generation and Pyruvate Formation
Following the investment phase, the glucose molecule is cleaved into two molecules of glyceraldehyde-3-phosphate (G3P). This marks the beginning of the energy-yielding reactions, where a net gain of ATP and NADH (nicotinamide adenine dinucleotide) occurs. Through a series of enzymatic reactions, including the action of glyceraldehyde-3-phosphate dehydrogenase and pyruvate kinase, two ATP molecules and two NADH molecules are generated per G3P molecule. This results in a net gain of 2 ATP and 2 NADH per glucose molecule. The final product of glycolysis is pyruvate, a three-carbon molecule.
Glycolysis Regulation: A Delicate Balance
The rate of glycolysis is meticulously regulated to meet the cell's energy demands. Key regulatory enzymes, such as phosphofructokinase, are sensitive to energy levels within the cell. High levels of ATP inhibit phosphofructokinase activity, slowing down glycolysis, while low ATP levels stimulate the enzyme, accelerating glucose breakdown. This finely tuned control ensures that glucose oxidation proceeds at an appropriate rate.
Stage 2: Pyruvate Oxidation – Transition to Mitochondria
Pyruvate, the product of glycolysis, doesn't directly enter the citric acid cycle. Instead, it undergoes a crucial transition step within the mitochondrial matrix. This process, known as pyruvate oxidation, involves the following key steps:
Decarboxylation: Carbon Dioxide Release
Pyruvate is first decarboxylated, meaning a carbon dioxide molecule is removed. This reaction, catalyzed by the pyruvate dehydrogenase complex, releases CO2 as a waste product.
Oxidation and Acetyl-CoA Formation
The remaining two-carbon fragment is oxidized, and a molecule of NADH is generated. This two-carbon unit is then attached to coenzyme A, forming acetyl-CoA, the crucial entry point into the citric acid cycle.
Irreversible Commitment: Stepping into the Cycle
The conversion of pyruvate to acetyl-CoA is an irreversible step, committing the carbon atoms of glucose to complete oxidation within the citric acid cycle.
Stage 3: Citric Acid Cycle (Krebs Cycle or TCA Cycle) – Central Metabolic Hub
The citric acid cycle, also known as the Krebs cycle or TCA (tricarboxylic acid) cycle, is a cyclical series of enzymatic reactions that occur in the mitochondrial matrix. This central metabolic hub plays a pivotal role in energy generation and metabolic interconnections. Each acetyl-CoA molecule entering the cycle undergoes a series of oxidations and decarboxylations, resulting in the generation of:
- 2 CO2 molecules: These are released as waste products.
- 3 NADH molecules: These carry high-energy electrons to the electron transport chain.
- 1 FADH2 molecule: (flavin adenine dinucleotide) Another electron carrier that contributes to ATP synthesis.
- 1 GTP molecule (guanosine triphosphate): This is readily converted to ATP, providing a direct source of energy.
Citric Acid Cycle Enzymes: A Choreographed Dance
The citric acid cycle involves a series of specific enzymes, each catalyzing a distinct reaction. The coordinated action of these enzymes ensures the efficient and regulated flow of metabolites through the cycle. Key enzymes include citrate synthase, isocitrate dehydrogenase, and α-ketoglutarate dehydrogenase. These enzymes are often regulatory points, sensitive to energy levels within the cell.
Citric Acid Cycle Regulation: Meeting Cellular Needs
Similar to glycolysis, the citric acid cycle's activity is tightly regulated to meet the cell's energy demands. The availability of substrates, such as acetyl-CoA, and the levels of ATP and NADH influence the activity of key enzymes, ensuring a balanced and efficient metabolic flux.
Stage 4: Oxidative Phosphorylation – The Powerhouse of the Cell
Oxidative phosphorylation, the final stage of glucose oxidation, occurs in the inner mitochondrial membrane. This process harnesses the energy stored in NADH and FADH2 to generate a large quantity of ATP via chemiosmosis.
Electron Transport Chain: A Cascade of Redox Reactions
NADH and FADH2 donate their high-energy electrons to a series of protein complexes embedded within the inner mitochondrial membrane, collectively known as the electron transport chain (ETC). As electrons move down the ETC, energy is released and used to pump protons (H+) from the mitochondrial matrix across the inner membrane into the intermembrane space. This creates a proton gradient, a difference in proton concentration across the membrane.
Chemiosmosis: ATP Synthase and the Proton Motive Force
The proton gradient established by the ETC represents potential energy, also known as the proton motive force. This force drives protons back across the inner mitochondrial membrane through a protein complex called ATP synthase. As protons flow through ATP synthase, it rotates, causing a conformational change that leads to the synthesis of ATP from ADP and inorganic phosphate (Pi). This remarkable process is known as chemiosmosis.
Oxygen: The Final Electron Acceptor
Oxygen plays a critical role in oxidative phosphorylation as the final electron acceptor at the end of the ETC. It combines with protons and electrons to form water, completing the electron transport chain and maintaining the flow of electrons. Without oxygen, the ETC would become blocked, halting ATP production.
The Overall Yield of Glucose Oxidation: A Summary
The complete oxidative breakdown of one glucose molecule yields a substantial amount of energy:
- Around 30-32 ATP molecules: The exact number varies slightly depending on the efficiency of the shuttle systems transferring electrons from NADH produced in the cytoplasm to the mitochondria.
- 6 CO2 molecules: These are waste products of cellular respiration.
- 6 H2O molecules: These are formed when oxygen acts as the final electron acceptor.
Beyond Glucose: Other Fuel Sources
While glucose is the primary fuel source for cellular respiration, other molecules can also be oxidized to generate ATP. These include fatty acids, amino acids, and ketone bodies. These alternative fuel sources often enter the metabolic pathway at different points, contributing to the overall energy production of the cell.
Cellular Respiration and Human Health: Implications and Disorders
Defects in any stage of cellular respiration can have significant implications for human health. Genetic disorders affecting mitochondrial function can lead to a variety of debilitating conditions. Furthermore, understanding cellular respiration is crucial for comprehending metabolic diseases such as diabetes, where glucose metabolism is impaired.
Conclusion: A Symphony of Biochemical Reactions
The complete oxidative breakdown of glucose is a remarkable feat of biochemical engineering. The intricate interplay of enzymatic reactions, electron carriers, and proton gradients results in the efficient conversion of chemical energy stored within glucose into readily usable ATP. This energy fuels virtually all cellular processes, underpinning the very essence of life. Understanding this fundamental process provides invaluable insights into cellular metabolism and the complexities of living organisms.
Latest Posts
Latest Posts
-
Valence Bond Theory Is Based On The Idea That
May 11, 2025
-
Properties Of Water That Make It Important To Life
May 11, 2025
-
A Molecule Is Said To Be Organic If It Contains
May 11, 2025
-
Estimate The Change In Enthalpy And Entropy When Liquid Ammonia
May 11, 2025
-
What Is Measured In An Experiment
May 11, 2025
Related Post
Thank you for visiting our website which covers about Complete Oxidative Breakdown Of Glucose Results In . We hope the information provided has been useful to you. Feel free to contact us if you have any questions or need further assistance. See you next time and don't miss to bookmark.