Complete The Model Of The Lactic Acid Fermentation Process.
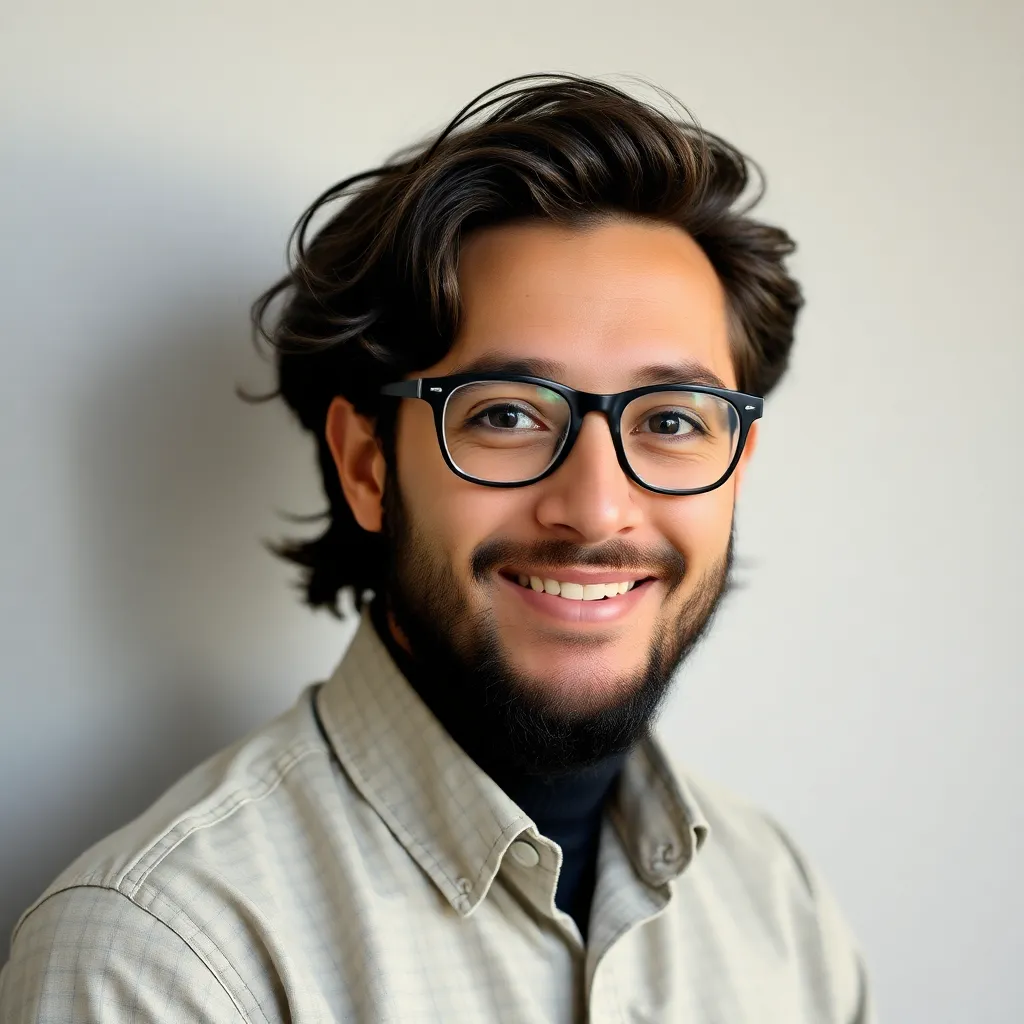
Muz Play
Apr 26, 2025 · 7 min read

Table of Contents
A Complete Model of the Lactic Acid Fermentation Process
Lactic acid fermentation, a cornerstone of anaerobic metabolism, is a vital process across numerous biological systems, from yogurt production to muscle function. This process, while seemingly simple, involves a complex interplay of enzymatic reactions, environmental factors, and regulatory mechanisms. This article delves deep into a complete model of lactic acid fermentation, exploring its intricacies from the initial substrate to the final product and covering key aspects relevant to various applications.
Understanding the Fundamentals of Lactic Acid Fermentation
Lactic acid fermentation is an anaerobic metabolic pathway that converts glucose or other six-carbon sugars into cellular energy (ATP) and lactic acid. Unlike aerobic respiration, which utilizes oxygen as a final electron acceptor, lactic acid fermentation relies on an endogenous electron acceptor, pyruvate, thereby generating a less efficient but crucial energy source in oxygen-deprived environments.
The Core Reaction: From Glucose to Lactic Acid
The core of lactic acid fermentation hinges on the glycolytic pathway. This pathway, a near-universal process across all living organisms, breaks down glucose into two molecules of pyruvate. This breakdown yields a net gain of two ATP molecules through substrate-level phosphorylation—a direct transfer of a phosphate group from a phosphorylated intermediate to ADP, forming ATP without the involvement of an electron transport chain.
The equation summarizing this process is:
C₆H₁₂O₆ → 2 CH₃CHOHCOOH + 2 ATP
Where:
- C₆H₁₂O₆ represents glucose
- CH₃CHOHCOOH represents lactic acid
- ATP represents adenosine triphosphate (the energy currency of cells)
Crucially, the production of lactic acid is not merely a byproduct but a vital step in regenerating NAD+, a critical coenzyme necessary for the continuation of glycolysis. Without the reduction of pyruvate to lactate, the glycolytic pathway would halt due to the depletion of NAD+, severely impacting ATP production.
Key Enzymes in the Process
Several key enzymes orchestrate the conversion of glucose to lactic acid:
- Hexokinase: Phosphorylates glucose, trapping it within the cell and initiating glycolysis.
- Phosphofructokinase (PFK): A rate-limiting enzyme in glycolysis; it catalyzes the phosphorylation of fructose-6-phosphate, committing the glucose molecule to complete glycolysis.
- Glyceraldehyde-3-phosphate dehydrogenase (GAPDH): Oxidizes glyceraldehyde-3-phosphate, producing NADH and a high-energy phosphate compound. This step is pivotal in NADH generation, crucial for the subsequent reduction of pyruvate.
- Lactate dehydrogenase (LDH): Catalyzes the final step, reducing pyruvate to lactic acid using the NADH produced earlier. This regeneration of NAD+ is essential for the continued operation of glycolysis.
The intricate regulation of these enzymes, often through allosteric modulation and feedback inhibition, finely tunes the rate of lactic acid fermentation in response to cellular energy demands and environmental conditions.
Factors Influencing Lactic Acid Fermentation
The efficiency and rate of lactic acid fermentation are not solely determined by the enzymatic machinery. Several environmental and biological factors significantly influence the process:
Substrate Availability
The type and concentration of the fermentable substrate (typically glucose or other hexoses) directly impact the yield of lactic acid and ATP. Higher substrate concentrations generally lead to higher fermentation rates until a saturation point is reached. Moreover, the availability of alternative carbon sources can also influence fermentation, especially in mixed microbial communities.
pH and Temperature
Optimal pH and temperature are critical for enzyme activity. The optimal pH range for most lactic acid bacteria is slightly acidic (around pH 5-6). Variations from this range can affect enzyme activity, slowing down or inhibiting fermentation. Similarly, temperature affects the reaction rates of enzymes; temperatures outside the optimal range can lead to decreased activity or enzyme denaturation.
Oxygen Availability (or Lack Thereof)
While labeled as "anaerobic," it's crucial to understand that lactic acid fermentation occurs optimally under strict anaerobic conditions. Although some lactic acid bacteria exhibit limited tolerance to oxygen, the presence of even small amounts of oxygen can inhibit the process, shifting the metabolism toward aerobic respiration if possible. This oxygen sensitivity is due to the oxygen's inhibitory effects on key enzymes in the glycolytic pathway and pyruvate reduction.
Microbial Community Composition
In many natural and industrial settings, lactic acid fermentation is not conducted by a single species but a diverse community of microorganisms. The interactions within these communities, including competition for substrates and the production of inhibitory byproducts, can significantly influence the overall fermentation process.
Different Types of Lactic Acid Fermentation
The process of lactic acid fermentation isn't monolithic. Two main pathways exist, each yielding slightly different products:
Homolactic Fermentation
In homolactic fermentation, glucose is almost entirely converted into lactic acid. This is the dominant pathway for many lactic acid bacteria, yielding two moles of lactic acid per mole of glucose. This pathway is highly efficient in ATP production relative to other fermentative pathways.
Heterolactic Fermentation
Heterolactic fermentation results in a more diverse range of products. Besides lactic acid, it yields other metabolites like ethanol, acetic acid, and carbon dioxide. This pathway involves a pentose phosphate pathway in addition to glycolysis, leading to the production of a variety of metabolites.
Applications of Lactic Acid Fermentation
Lactic acid fermentation plays a crucial role in various industries and biological systems:
Food Production
- Dairy Products: Yogurt, cheese, and other fermented dairy products rely heavily on lactic acid fermentation for their characteristic flavors, textures, and preservation. Lactic acid bacteria are used as starter cultures to produce these foods.
- Silage Production: Lactic acid fermentation preserves animal fodder by converting sugars in plants into lactic acid, creating an acidic environment that inhibits the growth of spoilage microorganisms.
- Pickling and Fermenting Vegetables: Processes like sauerkraut and kimchi utilize lactic acid bacteria to ferment vegetables, resulting in characteristic flavors and improved preservation.
- Bakery Products: The use of sourdough starters, which involve lactic acid fermentation, contributes to flavor development and textural changes in bread.
Industrial Applications
- Bioplastics: Lactic acid is a key building block for biodegradable plastics (polylactic acid or PLA), making it a sustainable alternative to petroleum-based plastics.
- Biomedical Applications: Lactic acid and its derivatives are used in various biomedical applications, such as wound healing, drug delivery, and tissue engineering.
Biological Significance
- Muscle Metabolism: During intense exercise when oxygen supply is limited, muscle cells undergo lactic acid fermentation to generate ATP. The accumulation of lactic acid contributes to muscle fatigue.
- Microbial Ecology: Lactic acid fermentation plays a vital role in various microbial ecosystems, influencing nutrient cycling and community dynamics.
Modeling Lactic Acid Fermentation: A Systems Biology Approach
A comprehensive understanding of lactic acid fermentation necessitates a systems biology approach. This involves integrating knowledge from different levels, including genomics, transcriptomics, proteomics, and metabolomics, to create a holistic model that captures the dynamic interplay of genes, proteins, and metabolites. Such a model can be computationally simulated to predict fermentation outcomes under various conditions, optimizing the process for industrial applications.
This type of modeling can help predict:
- Optimal fermentation conditions: Identifying the ideal temperature, pH, and substrate concentrations to maximize lactic acid production.
- Strain selection: Choosing the best microbial strains for specific applications based on their metabolic characteristics and fermentation efficiency.
- Metabolic engineering: Developing genetically modified strains with improved fermentation performance.
- Process control: Designing and implementing strategies to control and monitor fermentation processes, maintaining optimal conditions for efficient production.
Future Directions and Challenges
While significant progress has been made in understanding lactic acid fermentation, several challenges remain:
- Improving fermentation efficiency: Research continues to focus on improving the yield and rate of lactic acid production by optimizing fermentation conditions and engineering microbial strains.
- Developing sustainable fermentation processes: Reducing the environmental impact of lactic acid production is a priority, involving strategies like utilizing renewable energy sources and minimizing waste generation.
- Understanding complex microbial communities: Investigating the dynamics of microbial communities involved in lactic acid fermentation is crucial for optimizing mixed-culture fermentation processes.
- Integrating omics data for more accurate modeling: Integrating data from various omics technologies is vital for creating sophisticated models that accurately capture the complexities of lactic acid fermentation.
In conclusion, lactic acid fermentation is a multifaceted process with significant implications across diverse fields. A thorough understanding of its intricate mechanisms, coupled with advanced modeling techniques, will propel further advancements in its applications, fostering sustainable and efficient production of valuable products and improving our understanding of biological systems. The ongoing research in this area promises exciting developments, paving the way for innovative solutions in food production, biomaterials, and biotechnology.
Latest Posts
Latest Posts
-
When Cations And Anions Meet They
Apr 27, 2025
-
Does Oxygen And Fluorine Form An Ionic Compound
Apr 27, 2025
-
Common Stockholders Have The Right To
Apr 27, 2025
-
What Is Lemon Juice Ph Level
Apr 27, 2025
-
What Are Two Subatomic Particles Found In The Nucleus
Apr 27, 2025
Related Post
Thank you for visiting our website which covers about Complete The Model Of The Lactic Acid Fermentation Process. . We hope the information provided has been useful to you. Feel free to contact us if you have any questions or need further assistance. See you next time and don't miss to bookmark.