Consider The Sn2 Reaction Between 1-bromo-2-methylpropane And Methoxide
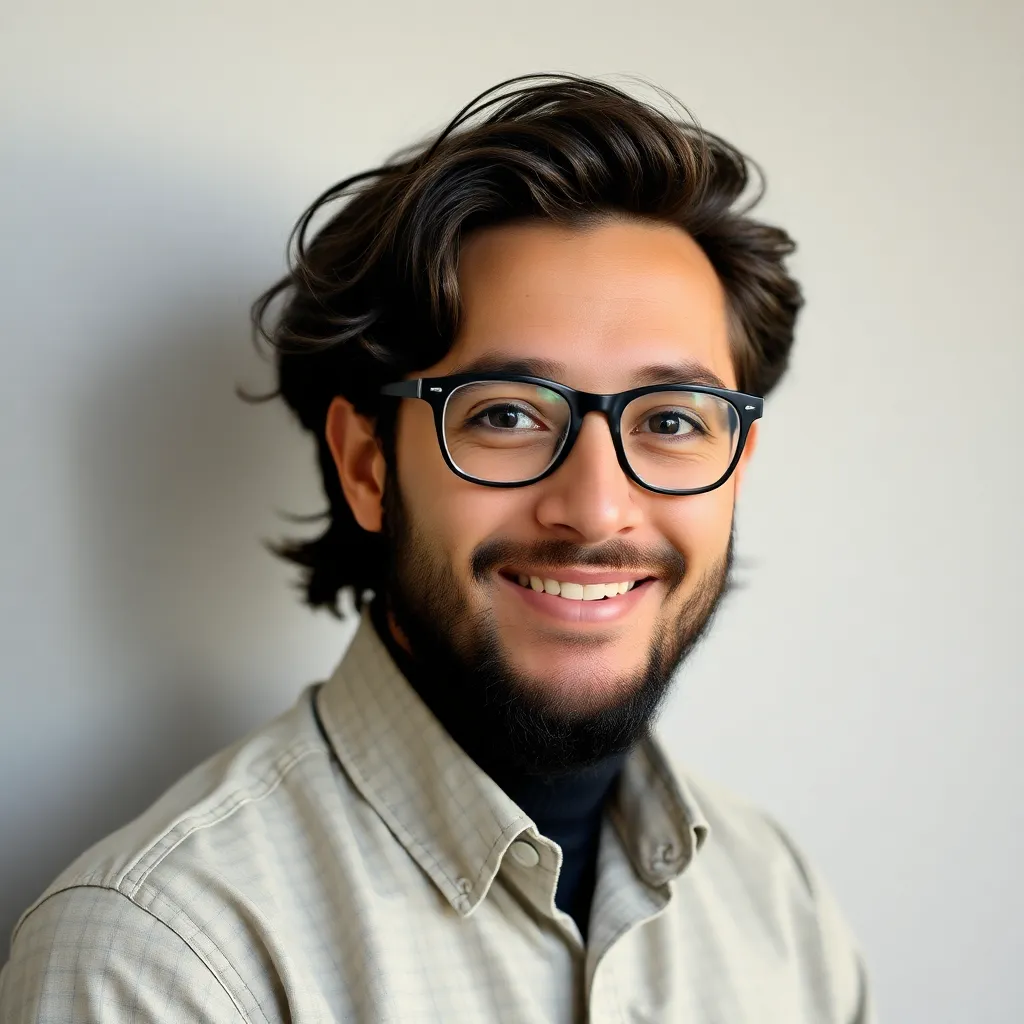
Muz Play
May 10, 2025 · 5 min read
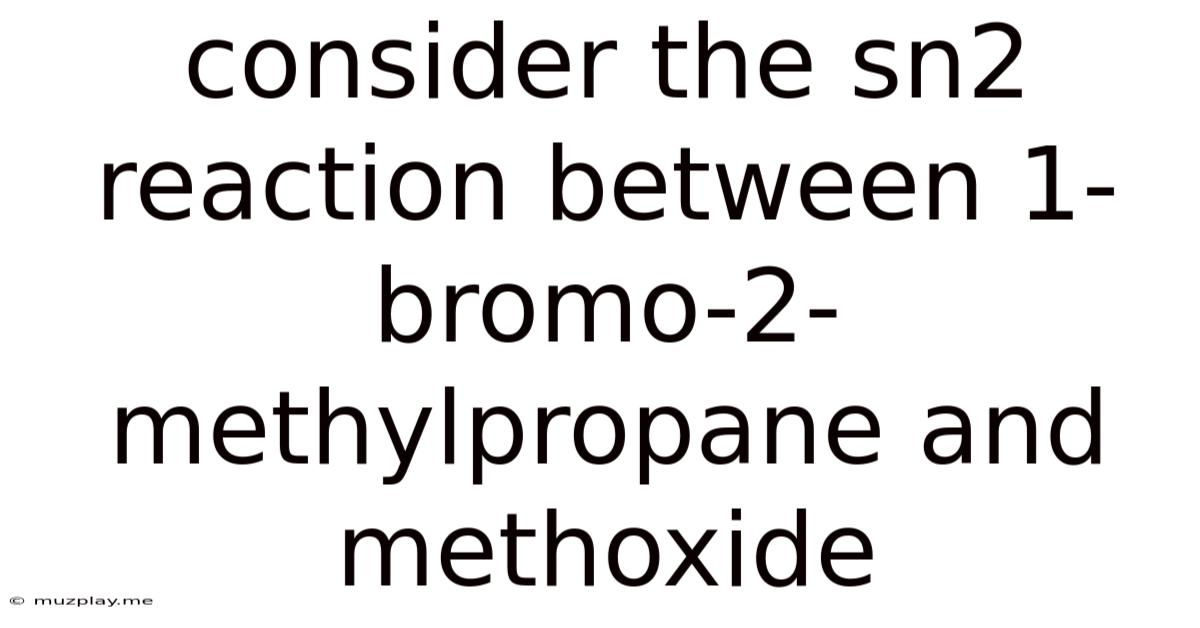
Table of Contents
Considering the SN2 Reaction Between 1-Bromo-2-methylpropane and Methoxide
The SN2 reaction, a fundamental concept in organic chemistry, represents a powerful nucleophilic substitution mechanism. This article delves into the specifics of the SN2 reaction between 1-bromo-2-methylpropane (also known as isobutyl bromide) and methoxide (CH₃O⁻), exploring the reaction mechanism, factors influencing its rate, stereochemistry, and potential side reactions. We'll also examine the practical implications and applications of this reaction.
Understanding the SN2 Reaction Mechanism
The SN2 reaction, meaning bimolecular nucleophilic substitution, is a concerted process where the nucleophile attacks the substrate simultaneously as the leaving group departs. This contrasts with SN1 reactions, which proceed via a two-step mechanism involving a carbocation intermediate.
In the reaction between 1-bromo-2-methylpropane and methoxide, methoxide acts as the nucleophile, attacking the carbon atom bearing the bromine atom. Bromine, being a good leaving group, departs simultaneously. This leads to the inversion of configuration at the stereocenter, a hallmark of the SN2 mechanism.
Mechanism Diagram:
(Imagine a diagram here showing the backside attack of methoxide on the carbon atom bonded to bromine in 1-bromo-2-methylpropane, with the simultaneous departure of bromide. Arrows should illustrate the movement of electrons.)
Key Features of the SN2 Mechanism:
- Concerted Mechanism: The nucleophilic attack and the leaving group departure occur in one single step.
- Backside Attack: The nucleophile attacks the carbon atom from the opposite side of the leaving group. This leads to inversion of stereochemistry at the chiral center.
- Bimolecular: The rate of the reaction depends on the concentrations of both the substrate and the nucleophile (rate = k[substrate][nucleophile]).
- Strong Nucleophile Required: A strong nucleophile is essential for a successful SN2 reaction. Weak nucleophiles are less likely to successfully displace the leaving group.
Stereospecificity and the Walden Inversion
A crucial aspect of the SN2 reaction is its stereospecificity. Because of the backside attack, the reaction results in Walden inversion, also known as inversion of configuration. If the starting material is chiral, the product will have the opposite stereochemistry. This is a significant diagnostic tool in identifying SN2 reactions.
Let's consider a specific example. If we start with (R)-1-bromo-2-methylpropane, the SN2 reaction with methoxide will yield (S)-2-methyl-1-methoxypropane. The configuration at the chiral carbon has been inverted.
Factors Influencing the Rate of the SN2 Reaction
Several factors significantly influence the rate of an SN2 reaction. These include:
Nature of the Substrate:
-
Steric Hindrance: Bulky substituents around the carbon atom bearing the leaving group hinder the approach of the nucleophile. 1-Bromo-2-methylpropane has a moderately hindered carbon due to the presence of the methyl group. This steric hindrance reduces the rate of the SN2 reaction compared to a less substituted substrate like 1-bromopropane. Highly branched substrates often undergo SN1 reactions preferentially.
-
Leaving Group Ability: Good leaving groups facilitate the SN2 reaction. Bromide (Br⁻) is an excellent leaving group, making this reaction feasible. Weaker leaving groups, such as hydroxide (OH⁻), would significantly slow down or prevent the reaction.
Nature of the Nucleophile:
-
Nucleophilicity: Stronger nucleophiles react faster in SN2 reactions. Methoxide (CH₃O⁻) is a relatively strong nucleophile, making the reaction proceed at a reasonable rate. The nucleophilicity of anionic nucleophiles often increases down a group in the periodic table (e.g., I⁻ > Br⁻ > Cl⁻). The solvent also plays a role here, as polar protic solvents can solvate the nucleophile and reduce its effectiveness.
-
Concentration: As mentioned previously, the rate of the SN2 reaction is directly proportional to the concentration of the nucleophile. Increasing the concentration of methoxide will increase the reaction rate.
Solvent Effects:
-
Polar Aprotic Solvents: These solvents are ideal for SN2 reactions because they solvate the cation (in this case, Na⁺ from the methoxide source) but not the nucleophile, leaving the nucleophile relatively "naked" and highly reactive. Examples include dimethyl sulfoxide (DMSO) and acetone.
-
Polar Protic Solvents: These solvents solvate both the cation and the nucleophile, reducing the nucleophile's reactivity and thus slowing down the SN2 reaction. Water and methanol are examples of polar protic solvents.
Potential Side Reactions
While the SN2 reaction is the dominant pathway under appropriate conditions, other reactions could occur as side reactions. These include:
-
Elimination Reactions: At higher temperatures or with stronger bases, elimination reactions (E2) can compete with the SN2 reaction. The E2 reaction would yield alkenes as products. In the case of 1-bromo-2-methylpropane, the major product of E2 elimination would be 2-methylpropene (isobutene).
-
SN1 Reactions: While less likely due to the relatively stable nature of the substrate and the use of strong nucleophile, SN1 reactions could potentially occur under certain conditions (e.g., a polar protic solvent).
Practical Applications and Importance
The SN2 reaction, exemplified by the reaction between 1-bromo-2-methylpropane and methoxide, has numerous applications in organic synthesis:
-
Alkylation of Alcohols: This particular reaction is an example of alkylation of an alcohol (methanol, which is the conjugate acid of methoxide). Alkylation is crucial in the synthesis of a wide range of organic molecules.
-
Synthesis of Ethers: The SN2 reaction is a common method for synthesizing ethers. The resulting 2-methyl-1-methoxypropane is an example of an ether.
-
Preparation of Other Functional Groups: SN2 reactions are foundational to many synthetic strategies, serving as building blocks for preparing complex molecules with various functional groups.
-
Pharmaceutical Industry: SN2 reactions are widely used in the pharmaceutical industry for the synthesis of various drugs and medicinal compounds.
Conclusion
The SN2 reaction between 1-bromo-2-methylpropane and methoxide offers a valuable case study for understanding the principles of nucleophilic substitution. The factors influencing the reaction rate, including steric hindrance, leaving group ability, nucleophilicity, and solvent effects, are crucial for controlling the reaction outcome. Understanding these factors allows chemists to design and optimize synthetic routes for the preparation of a wide range of valuable compounds. The stereochemical outcome, Walden inversion, provides a key diagnostic tool for confirming the SN2 mechanism. However, the possibility of competing side reactions, particularly E2 elimination, must be considered and managed through careful control of reaction conditions. Mastering the SN2 reaction is essential for any organic chemist, given its central role in synthesis and its widespread applications in various fields.
Latest Posts
Latest Posts
-
What Determines The Shape Of A Protein
May 10, 2025
-
At What Levels Do Biologists Study Life
May 10, 2025
-
What Is The Heat Of Formation Reaction For Sodium Chloride
May 10, 2025
-
How Do Row Operations Affect The Determinant
May 10, 2025
-
Accepted Value Of Density Of Aluminum
May 10, 2025
Related Post
Thank you for visiting our website which covers about Consider The Sn2 Reaction Between 1-bromo-2-methylpropane And Methoxide . We hope the information provided has been useful to you. Feel free to contact us if you have any questions or need further assistance. See you next time and don't miss to bookmark.