Definition Of Time Interval In Physics
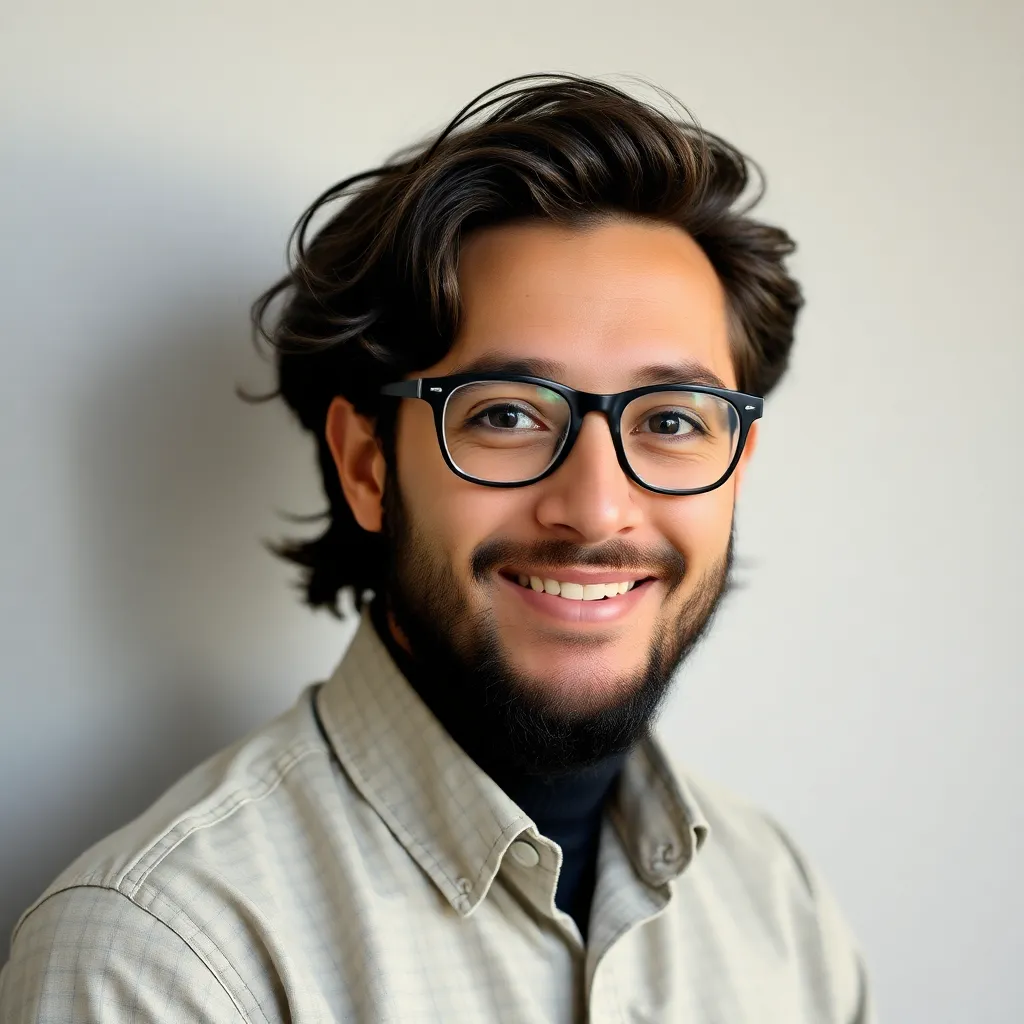
Muz Play
Apr 27, 2025 · 7 min read

Table of Contents
Delving Deep into Time Intervals in Physics: A Comprehensive Guide
The concept of a time interval is fundamental to physics, underpinning our understanding of motion, change, and the very fabric of the universe. While seemingly intuitive in everyday life – the time between two events – its precise definition and implications within the rigorous framework of physics require closer examination. This article will explore the definition of a time interval, its measurement, its relationship to other physical quantities, and its significance across various branches of physics, from classical mechanics to relativity.
What is a Time Interval in Physics?
In physics, a time interval is defined as the duration between two distinct events. These events can be anything from the start and stop of a stopwatch to the emission and absorption of a photon. Crucially, it’s not simply a point in time, but the difference between two temporal points. This difference is often denoted by Δt (delta t), signifying a change in time. The precise measurement of this interval depends heavily on the chosen reference frame and the precision of the measuring instrument.
Defining Events: The Foundation of Time Intervals
The accuracy of a time interval measurement hinges entirely on the clarity and precision of defining the events that mark its beginning and end. These events need to be objectively identifiable and repeatable. For instance, in measuring the period of a pendulum, the events could be defined as consecutive passages of the bob through its lowest point. In tracking the decay of a radioactive isotope, the events might be defined as the detection of emitted particles. The clearer the definition, the more reliable the measurement of the time interval.
Measuring Time Intervals: From Sundials to Atomic Clocks
The methods used to measure time intervals have evolved dramatically throughout history. Early methods relied on periodic phenomena in nature, like the Earth's rotation (sundials) or the rhythmic dripping of water (water clocks). These methods, while useful, lacked precision and were susceptible to various environmental influences.
The development of more sophisticated timekeeping devices, such as mechanical clocks and later, quartz crystal oscillators, significantly improved accuracy. However, the most precise time interval measurements today are achieved using atomic clocks. These clocks leverage the incredibly precise and stable oscillations of atoms, such as cesium-133, to define a second with astonishing accuracy. The International System of Units (SI) defines the second based on the transitions within cesium-133 atoms. This standardization ensures consistency across scientific measurements worldwide.
Accuracy and Precision in Time Interval Measurement
While atomic clocks offer remarkable precision, even these instruments are subject to limitations. Factors like gravitational fields and temperature fluctuations can subtly affect the frequency of atomic oscillations, introducing small errors into the time interval measurement. Scientists are continually working to improve the accuracy and stability of atomic clocks to refine measurements in diverse fields, from GPS technology to fundamental physics research.
Time Intervals and Other Physical Quantities: Intertwined Relationships
Time intervals are intimately linked with other fundamental physical quantities, most notably:
1. Velocity and Acceleration:
In classical mechanics, the concept of velocity is defined as the change in displacement (distance) over a change in time (Δt). Similarly, acceleration is defined as the change in velocity over a change in time (Δt). These relationships are crucial for analyzing motion and predicting the trajectory of objects. For example, calculating the average speed of a car involves dividing the distance traveled by the time interval taken.
2. Frequency and Period:
In oscillatory motion and wave phenomena, frequency (f) represents the number of cycles or oscillations per unit of time, while the period (T) represents the time interval for one complete cycle. The relationship between frequency and period is inversely proportional: f = 1/T. This relationship applies to diverse systems, including pendulums, springs, and electromagnetic waves.
3. Energy and Power:
The concept of power in physics relates the amount of energy transferred or work done to the time interval over which it occurs. Power is defined as the rate of energy transfer, expressed as energy divided by the time interval (Δt). For instance, the power of an engine is determined by the amount of work it does per unit time.
4. Radioactive Decay and Half-Life:
In nuclear physics, the half-life of a radioactive isotope refers to the time interval it takes for half of the atoms in a sample to undergo radioactive decay. This is a probabilistic process, but the half-life is a characteristic property of the specific isotope. Accurate measurement of time intervals is crucial for dating artifacts using radiocarbon dating or for monitoring radiation levels in various applications.
Time Intervals in Different Branches of Physics
The concept of a time interval plays a crucial role in various branches of physics:
1. Classical Mechanics:
In classical mechanics, time is treated as an absolute and universal quantity. This means that the time interval between two events is the same for all observers, irrespective of their relative motion. This assumption simplifies the analysis of motion and interaction of objects but breaks down at very high speeds approaching the speed of light.
2. Special Relativity:
Einstein's theory of special relativity revolutionized our understanding of time. It postulates that the time interval between two events depends on the relative motion of the observer. This phenomenon is known as time dilation, where moving clocks run slower relative to stationary clocks. This effect is only significant at speeds approaching the speed of light and has been experimentally verified.
3. General Relativity:
General relativity extends special relativity to include gravity. It describes gravity not as a force but as a curvature of spacetime caused by mass and energy. In general relativity, the time interval between two events is affected not only by relative motion but also by the strength of the gravitational field. This means that time runs slower in stronger gravitational fields – a phenomenon known as gravitational time dilation. GPS technology requires precise accounting for both special and general relativistic effects on time intervals to maintain accurate positioning.
4. Quantum Mechanics:
In quantum mechanics, time is treated as a continuous parameter but its precise meaning and measurement are subject to debate. The time-energy uncertainty principle states that the precision with which both the energy and the time of an event can be known is limited. This principle has profound implications for understanding the evolution of quantum systems. The time it takes for a quantum system to transition between states is another significant consideration in quantum mechanics.
5. Cosmology:
In cosmology, the study of the universe's origin and evolution, the concept of a time interval is crucial for understanding the universe's age, the expansion rate of the universe, and the timeline of cosmic events, such as the formation of stars and galaxies.
Advanced Concepts Related to Time Intervals
Several more advanced concepts are intimately connected to the notion of time intervals:
1. Proper Time:
In special relativity, proper time is the time interval measured by a clock that is at rest relative to the event being observed. It represents the time experienced by an observer in their own reference frame.
2. Coordinate Time:
Coordinate time is a time coordinate used in a specific reference frame, often used in discussions of spacetime in relativity. It is not the same as proper time and is dependent on the chosen coordinate system.
3. Time Asymmetry:
The arrow of time refers to the observation that time seems to flow in one direction, from the past to the future. This is related to the second law of thermodynamics, which states that the entropy (disorder) of a closed system always increases over time. Understanding the asymmetry of time remains a challenge in physics.
Conclusion: The Enduring Importance of Time Intervals in Physics
The seemingly simple concept of a time interval underpins much of our understanding of the physical world. From the basic laws of motion to the complexities of relativity and quantum mechanics, the precise measurement and interpretation of time intervals are crucial for advancing our knowledge. As our technology improves and our understanding of the universe deepens, the ongoing refinement of time interval measurements will continue to be a cornerstone of scientific progress. Further research into the nature of time itself promises to unravel even deeper mysteries of the cosmos and refine our models of the universe's fundamental workings. The journey into understanding time intervals is far from over, and each new step unveils further complexities and exciting possibilities.
Latest Posts
Latest Posts
-
Compound That Produces Hydroxide Ions In Solution
Apr 28, 2025
-
Can A Mixture Be Physically Separated
Apr 28, 2025
-
Functionally A Gomphosis Is Categorized As A
Apr 28, 2025
-
Which Quarter Has The Smallest Spread Of Data
Apr 28, 2025
-
What Are The Units For The Spring Constant K
Apr 28, 2025
Related Post
Thank you for visiting our website which covers about Definition Of Time Interval In Physics . We hope the information provided has been useful to you. Feel free to contact us if you have any questions or need further assistance. See you next time and don't miss to bookmark.