Describe The Sliding Filament Model Of Muscle Contraction
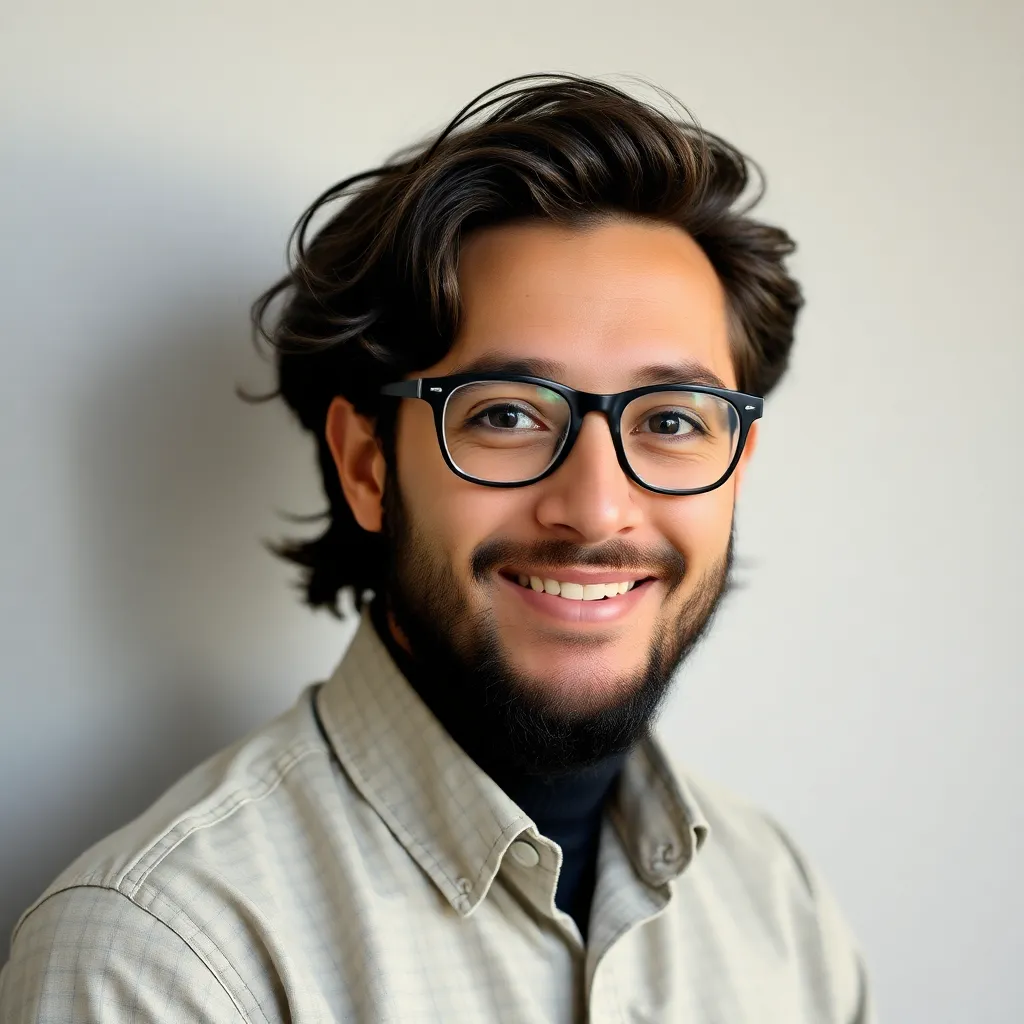
Muz Play
Mar 12, 2025 · 7 min read

Table of Contents
The Sliding Filament Model of Muscle Contraction: A Deep Dive
The human body is a marvel of engineering, capable of incredible feats of strength, agility, and endurance. At the heart of this capability lies the intricate machinery of muscle contraction. Understanding how muscles contract is crucial for comprehending movement, athletic performance, and various physiological processes. This article delves into the sliding filament model, the prevailing theory explaining muscle contraction at the molecular level. We'll explore the key players – actin, myosin, troponin, tropomyosin – and the precise steps involved in this remarkable process.
Understanding the Basic Components: Actin and Myosin
Before diving into the mechanics of the sliding filament model, let's establish a foundation by understanding the key protein players: actin and myosin. These two proteins are the workhorses of muscle contraction.
Actin: The Thin Filament
Actin filaments, also known as thin filaments, are long, helical polymers composed of globular actin (G-actin) monomers. These monomers link together to form a double-stranded helix. Associated with actin are two crucial regulatory proteins:
- Tropomyosin: A long, fibrous protein that winds around the actin filament, covering the myosin-binding sites on actin in a relaxed muscle.
- Troponin: A complex of three proteins (troponin I, troponin T, and troponin C) bound to tropomyosin. Troponin plays a crucial role in regulating muscle contraction by controlling the position of tropomyosin. Specifically, troponin C binds calcium ions, which is the trigger for muscle contraction.
Myosin: The Thick Filament
Myosin filaments, or thick filaments, are composed of hundreds of myosin molecules. Each myosin molecule is a dimer with two globular heads and a long tail. The tails intertwine to form the thick filament's core, while the heads project outward, forming cross-bridges that interact with actin filaments. These myosin heads possess ATPase activity, meaning they can break down ATP (adenosine triphosphate), the energy currency of cells, to power muscle contraction.
The Sliding Filament Model: A Step-by-Step Guide
The sliding filament model proposes that muscle contraction occurs through the sliding of actin filaments past myosin filaments, resulting in a shortening of the sarcomere, the basic contractile unit of muscle. This sliding is not a continuous process but rather a cyclical series of events involving the myosin heads. Let's break down this cycle:
-
ATP Hydrolysis and Myosin Head Activation: The process begins with an ATP molecule binding to the myosin head. This binding causes a conformational change in the myosin head, causing it to detach from the actin filament. ATP is then hydrolyzed into ADP (adenosine diphosphate) and inorganic phosphate (Pi), which remain bound to the myosin head. This hydrolysis energizes the myosin head, cocking it into a high-energy state.
-
Cross-Bridge Formation: The energized myosin head now binds to a myosin-binding site on the actin filament, forming a cross-bridge. This binding is facilitated by the movement of tropomyosin, which is regulated by the calcium-troponin interaction (explained below).
-
Power Stroke: Following cross-bridge formation, the myosin head undergoes a conformational change, releasing the inorganic phosphate (Pi). This change pivots the myosin head, generating a force that pulls the actin filament towards the center of the sarcomere – this is the power stroke.
-
ADP Release and Myosin Head Detachment: After the power stroke, ADP is released from the myosin head. The myosin head remains bound to the actin filament in a low-energy state.
-
ATP Binding and Cross-Bridge Detachment: A new ATP molecule binds to the myosin head, causing it to detach from the actin filament. The cycle can then repeat, provided calcium ions remain present.
The Role of Calcium Ions in Muscle Contraction
The presence of calcium ions (Ca2+) is absolutely critical for initiating and regulating muscle contraction. In a resting muscle, the concentration of Ca2+ in the cytoplasm is low, and tropomyosin blocks the myosin-binding sites on actin. This prevents cross-bridge formation and maintains the muscle in a relaxed state.
However, when a nerve impulse stimulates a muscle fiber, it triggers the release of Ca2+ from the sarcoplasmic reticulum (SR), a specialized intracellular calcium store. The increase in cytosolic Ca2+ concentration binds to troponin C, causing a conformational change in the troponin-tropomyosin complex. This change shifts tropomyosin, uncovering the myosin-binding sites on actin. This makes it possible for the myosin heads to interact with actin and initiate the sliding filament cycle, leading to muscle contraction.
When the nerve impulse ceases, Ca2+ is actively pumped back into the SR, reducing the cytosolic Ca2+ concentration. This causes tropomyosin to move back to its blocking position, preventing further cross-bridge formation, and thus relaxing the muscle.
Types of Muscle Contractions: Isometric and Isotonic
Muscle contractions can be categorized into two main types: isometric and isotonic.
Isometric Contractions
Isometric contractions occur when the muscle generates force without changing its length. Think of holding a heavy object in place – your muscles are working hard to maintain the position, but the length of the muscle doesn't change significantly.
Isotonic Contractions
Isotonic contractions involve a change in muscle length while maintaining a relatively constant tension. These are further divided into concentric and eccentric contractions:
- Concentric Contractions: The muscle shortens as it generates force. Examples include lifting a weight or flexing your bicep.
- Eccentric Contractions: The muscle lengthens while generating force. These are often involved in controlled movements, like lowering a weight slowly or extending your arm.
Factors Affecting Muscle Contraction
Several factors influence the strength and efficiency of muscle contraction:
-
Frequency of Stimulation: Rapid, repeated stimulation leads to a phenomenon called summation, where subsequent contractions build upon each other, producing a stronger overall contraction. High-frequency stimulation can result in tetanus, a sustained maximal contraction.
-
Number of Motor Units Recruited: Muscles are composed of many motor units, each containing a motor neuron and the muscle fibers it innervates. The strength of a contraction is determined by the number of motor units activated. Recruiting more motor units leads to a stronger contraction.
-
Length-Tension Relationship: The force a muscle can generate depends on its initial length. There is an optimal length at which the muscle can produce maximal force. If the muscle is too short or too stretched, the force generated will be reduced.
-
Fiber Type: Muscles contain different types of muscle fibers (Type I, Type IIa, Type IIx) that differ in their contractile properties, such as speed of contraction and fatigue resistance. The proportion of fiber types in a muscle influences its overall performance characteristics.
-
Energy Availability: Muscle contraction requires ATP. The availability of ATP determines the duration and strength of contraction. During prolonged activity, the muscle may switch to anaerobic metabolism, which leads to lactic acid accumulation and fatigue.
Muscle Fatigue and Recovery
Prolonged or intense muscle activity leads to muscle fatigue, a state of reduced capacity to produce force. Several factors contribute to muscle fatigue:
-
Depletion of Energy Stores: The depletion of ATP and glycogen reduces the ability of the muscle to sustain contraction.
-
Accumulation of Metabolic Byproducts: The build-up of lactic acid and inorganic phosphate can interfere with muscle function.
-
Electrolyte Imbalances: Changes in the concentration of ions such as potassium and sodium can affect muscle excitability and contractility.
-
Neural Factors: Changes in the central nervous system can also contribute to fatigue.
Muscle recovery involves restoring energy stores, removing metabolic byproducts, and replenishing electrolytes. Adequate rest, nutrition, and hydration are crucial for muscle recovery.
Clinical Relevance of the Sliding Filament Model
Understanding the sliding filament model has profound implications for various medical fields:
-
Muscle Disorders: The model helps explain the pathophysiology of many muscle disorders, such as muscular dystrophy, where defects in muscle proteins impair contraction.
-
Sports Medicine: Optimizing training regimens and recovery strategies relies on understanding the mechanics of muscle contraction and fatigue.
-
Rehabilitation: Rehabilitation programs are designed to restore muscle function following injury or illness, based on principles derived from the sliding filament model.
-
Pharmacology: Many drugs affecting muscle function, such as muscle relaxants and stimulants, work by modulating aspects of the sliding filament mechanism.
Conclusion
The sliding filament model provides a comprehensive explanation for the intricate process of muscle contraction. This elegant mechanism, involving the interplay of actin, myosin, troponin, tropomyosin, and calcium ions, enables movement, posture, and a multitude of other essential physiological functions. Continued research into the intricacies of muscle contraction promises further insights into health, disease, and human performance. A deeper understanding of this process is vital for advancements in various fields, from treating muscle disorders to optimizing athletic performance. The elegance and efficiency of the sliding filament model underscore the remarkable complexity and precision of biological systems.
Latest Posts
Latest Posts
-
Does Meat Spontaneously Generate Flies And Maggots
May 09, 2025
-
Amino Acids Are Built Around A Central
May 09, 2025
-
What Is The Total Magnification Of A Microscope
May 09, 2025
-
Is Smell A Chemical Or Physical Property
May 09, 2025
-
Cual Es El Proposito Del Gobierno
May 09, 2025
Related Post
Thank you for visiting our website which covers about Describe The Sliding Filament Model Of Muscle Contraction . We hope the information provided has been useful to you. Feel free to contact us if you have any questions or need further assistance. See you next time and don't miss to bookmark.