Determine The Direction That Each Of The Reactions Will Progress
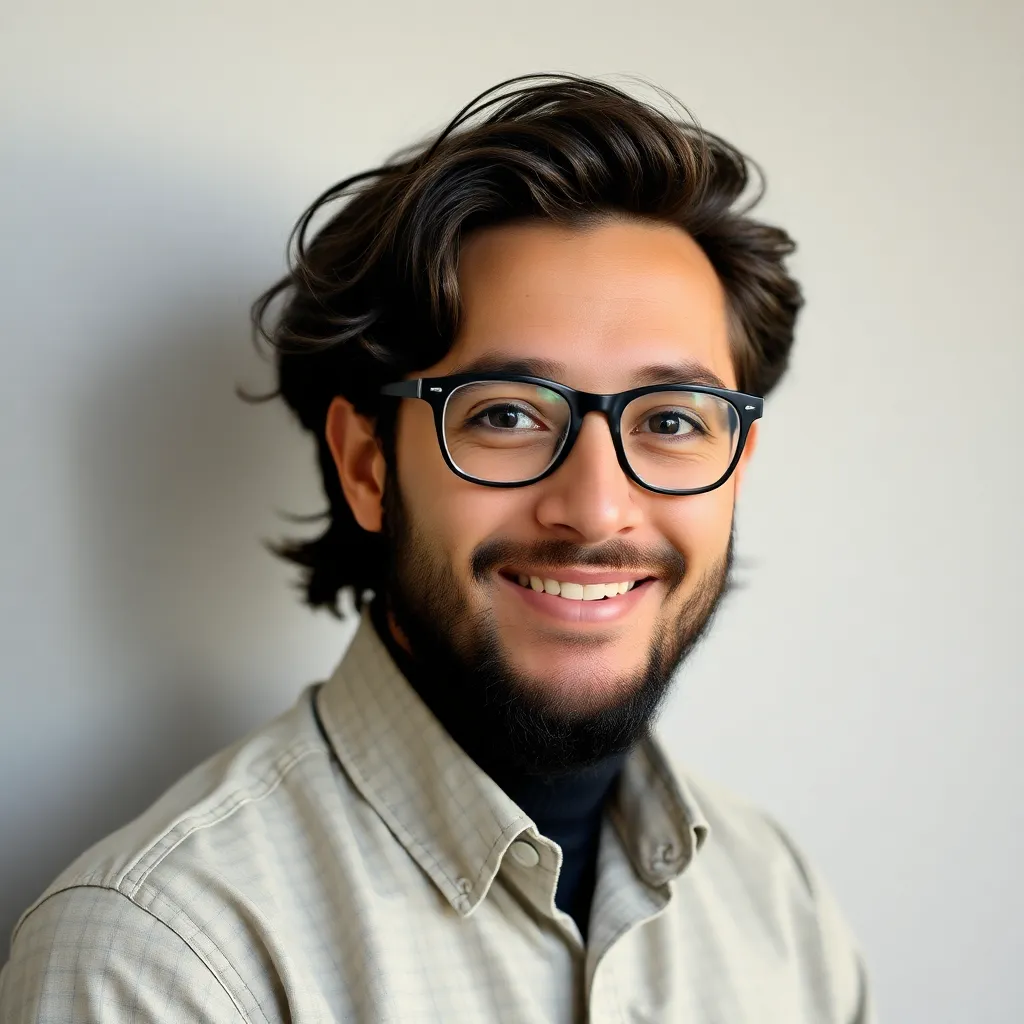
Muz Play
Apr 22, 2025 · 7 min read

Table of Contents
Determining the Direction of Chemical Reactions: A Comprehensive Guide
Predicting the direction a chemical reaction will proceed is a fundamental concept in chemistry. Understanding this allows us to design and optimize chemical processes, predict the outcome of reactions, and understand the equilibrium state of a system. This comprehensive guide explores various methods and principles used to determine the direction of a reaction, ranging from simple observations to complex thermodynamic calculations.
Understanding Equilibrium and the Reaction Quotient
Before delving into the methods, we need to establish a firm understanding of chemical equilibrium. A system is at equilibrium when the rates of the forward and reverse reactions are equal, resulting in no net change in the concentrations of reactants and products. This state is dynamic, meaning reactions continue to occur, but the overall composition remains constant.
The reaction quotient (Q) is a measure of the relative amounts of products and reactants at any given point in a reaction. It's calculated using the same expression as the equilibrium constant (K), but using the concentrations at a specific moment, not necessarily at equilibrium.
- Q < K: The reaction will proceed in the forward direction to reach equilibrium. This means more products will be formed.
- Q > K: The reaction will proceed in the reverse direction to reach equilibrium. This means more reactants will be formed.
- Q = K: The reaction is at equilibrium. There is no net change in the concentrations of reactants and products.
Methods for Determining Reaction Direction
Several methods can be employed to determine the direction a reaction will progress. These methods vary in complexity and the information required.
1. Using the Equilibrium Constant (K) and the Reaction Quotient (Q):
This is the most common and straightforward method. As discussed above, comparing Q and K allows us to predict the direction. The equilibrium constant (K) is a constant value specific to a reaction at a given temperature. It represents the ratio of products to reactants at equilibrium. A large K value indicates that the equilibrium favors the products, while a small K value indicates that the equilibrium favors the reactants.
Example: Consider the reaction A + B ⇌ C. If K = 10 and at a given point Q = 1, then Q < K, implying the reaction will proceed to the right (forming more C). Conversely, if Q = 100, then Q > K, and the reaction will shift to the left (forming more A and B).
2. Le Chatelier's Principle:
This principle states that if a change of condition is applied to a system in equilibrium, the system will shift in a direction that relieves the stress. These changes can include:
-
Changes in Concentration: Adding more reactant will shift the equilibrium to the right (favoring product formation), while adding more product will shift it to the left. Removing a reactant or product will have the opposite effect.
-
Changes in Pressure: Changes in pressure primarily affect gaseous reactions. Increasing pressure favors the side with fewer gas molecules, while decreasing pressure favors the side with more gas molecules.
-
Changes in Temperature: The effect of temperature changes depends on whether the reaction is exothermic (releases heat) or endothermic (absorbs heat). For exothermic reactions, increasing temperature shifts the equilibrium to the left, while decreasing temperature shifts it to the right. The opposite is true for endothermic reactions.
-
Changes in Volume: Changing the volume of the reaction vessel is similar to changing the pressure. Decreasing the volume increases the pressure, and increasing the volume decreases the pressure.
Example: Consider the exothermic reaction N₂(g) + 3H₂(g) ⇌ 2NH₃(g) + heat. Increasing the temperature will shift the equilibrium to the left, favoring the formation of N₂ and H₂. Increasing the pressure will shift the equilibrium to the right, favoring the formation of NH₃ because there are fewer gas molecules on the product side.
3. Gibbs Free Energy (ΔG):
Thermodynamics provides a powerful tool for predicting the spontaneity and direction of a reaction. The Gibbs Free Energy (ΔG) is a state function that determines whether a reaction will occur spontaneously under constant temperature and pressure.
- ΔG < 0: The reaction is spontaneous in the forward direction.
- ΔG > 0: The reaction is non-spontaneous in the forward direction; it will proceed in the reverse direction.
- ΔG = 0: The reaction is at equilibrium.
The Gibbs Free Energy change is related to the equilibrium constant (K) and the standard Gibbs Free Energy change (ΔG°) by the following equation:
ΔG = ΔG° + RTlnQ
where:
- R is the ideal gas constant
- T is the temperature in Kelvin
- Q is the reaction quotient
At equilibrium (Q = K and ΔG = 0), the equation simplifies to:
ΔG° = -RTlnK
This relationship allows us to calculate the equilibrium constant from the standard Gibbs Free Energy change and vice versa. A negative ΔG° indicates a reaction that favors product formation at equilibrium.
4. Standard Reduction Potentials (Electrochemical Reactions):
For electrochemical reactions (redox reactions), the direction of the reaction can be predicted by comparing the standard reduction potentials of the half-reactions. The reaction will proceed spontaneously in the direction that results in a positive cell potential (E°cell). The cell potential is calculated by subtracting the standard reduction potential of the anode (oxidation half-reaction) from the standard reduction potential of the cathode (reduction half-reaction):
E°cell = E°cathode - E°anode
A positive E°cell indicates a spontaneous reaction.
5. Qualitative Observations and Experimental Data:
Sometimes, simply observing the reaction can provide clues about its direction. For example, if a precipitate forms, it indicates that the reaction is proceeding in the direction that produces the precipitate. Similarly, the evolution of a gas or a significant color change can provide qualitative information about the reaction's progress. Experimental data, such as measuring the concentrations of reactants and products over time, can also be used to determine the direction and the rate of the reaction.
Factors Influencing Reaction Direction: A Deeper Dive
The direction of a chemical reaction is a complex interplay of several factors. While we've discussed the primary methods above, understanding these nuances is crucial for accurate predictions.
1. Temperature Dependence: The temperature dependence of the equilibrium constant (K) is described by the Van't Hoff equation:
d(lnK)/dT = ΔH°/RT²
where ΔH° is the standard enthalpy change of the reaction. This equation shows how the equilibrium constant changes with temperature, allowing for more precise predictions under varying conditions.
2. Concentration Effects: The concentrations of reactants and products significantly impact the reaction quotient (Q), directly influencing the direction of the reaction towards equilibrium. This is particularly important in industrial processes where manipulating concentrations is a key control mechanism.
3. Pressure Effects (Gas-Phase Reactions): The pressure exerted on a gaseous system impacts the equilibrium position, especially when the number of gas molecules on either side of the reaction differs. Increasing pressure favors the side with fewer gas molecules, and vice versa.
4. Catalyst Effects: While catalysts accelerate the rate of reaction, they do not alter the equilibrium position. They equally enhance the rates of both forward and reverse reactions, allowing the system to reach equilibrium faster.
Applications and Importance
The ability to predict the direction of chemical reactions has wide-ranging applications across various scientific and industrial fields.
-
Chemical Engineering: Optimizing industrial processes relies heavily on understanding and manipulating reaction equilibrium to maximize product yield and minimize waste.
-
Environmental Science: Predicting the fate of pollutants and understanding their environmental impact necessitates the ability to predict the direction of reactions involving these substances.
-
Biochemistry: Metabolic pathways in living organisms involve a series of interconnected reactions. Understanding the direction of these reactions is fundamental to understanding biological processes.
-
Materials Science: Designing new materials often involves controlling chemical reactions to produce specific structures and properties.
Conclusion
Determining the direction of a chemical reaction is a crucial skill in chemistry. This guide has presented various methods, from simple comparisons of Q and K to the application of thermodynamic principles and electrochemical considerations. Understanding these concepts allows for accurate predictions, enabling advancements in numerous fields. Remember that predicting the direction is not solely about calculations; it's also about integrating qualitative observations and understanding the underlying principles governing equilibrium and spontaneity. By mastering these techniques, one gains a deeper understanding of the dynamic world of chemical transformations.
Latest Posts
Latest Posts
-
Define Total Internal Reflection In Physics
Apr 22, 2025
-
Table 10 1 Selected Muscle Origins Insertions And Actions
Apr 22, 2025
-
What Does The Mouth Of The Cnidarian Open Into
Apr 22, 2025
-
In A Hypotonic Solution An Animal Cell Will
Apr 22, 2025
-
How Many Oxygen Atoms Are In A Molecule Of Water
Apr 22, 2025
Related Post
Thank you for visiting our website which covers about Determine The Direction That Each Of The Reactions Will Progress . We hope the information provided has been useful to you. Feel free to contact us if you have any questions or need further assistance. See you next time and don't miss to bookmark.