Difference Between Isothermal And Adiabatic Process
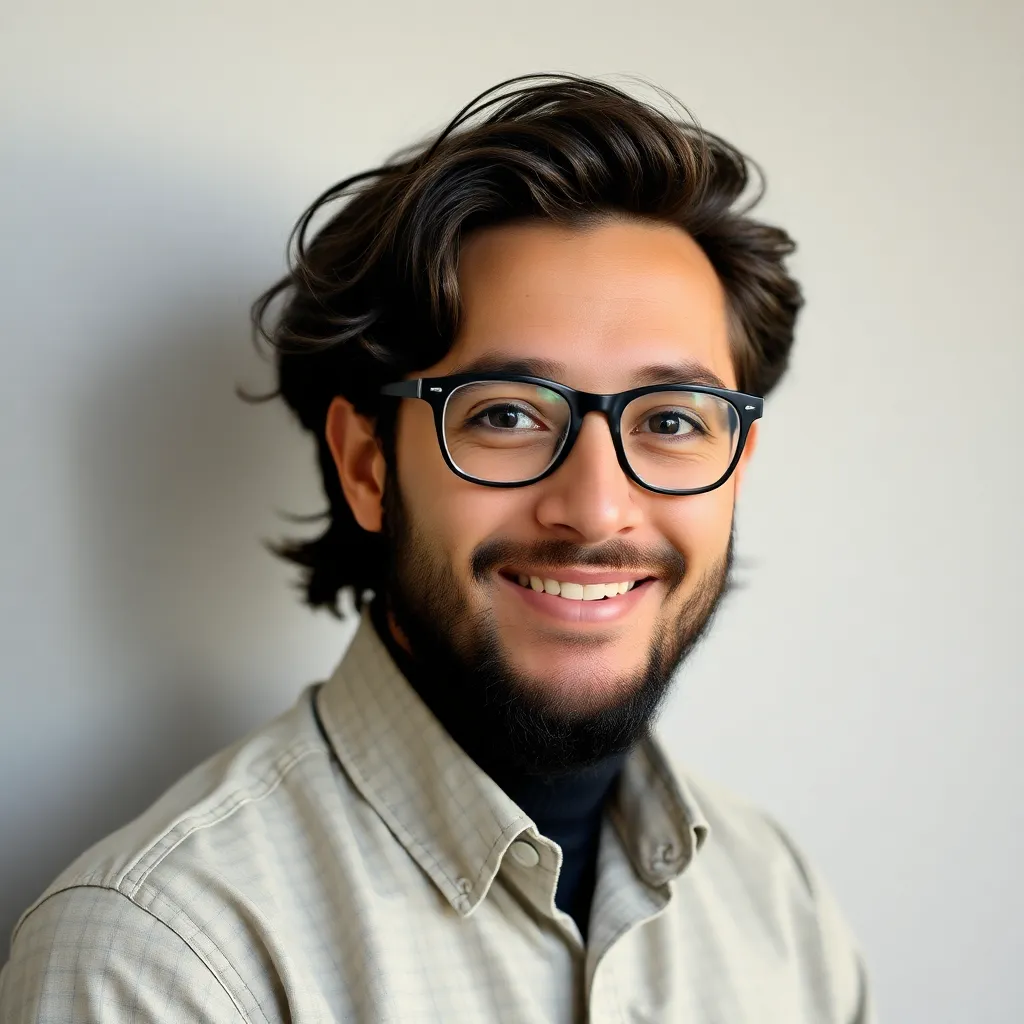
Muz Play
May 10, 2025 · 6 min read
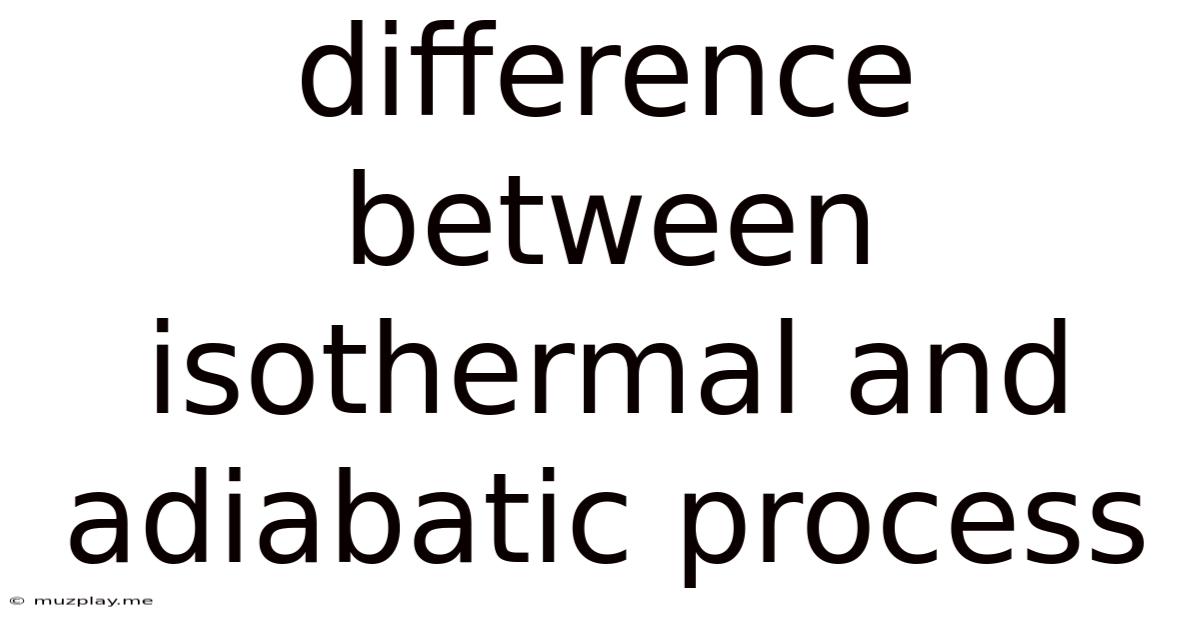
Table of Contents
The Fundamental Difference Between Isothermal and Adiabatic Processes
Understanding thermodynamic processes is crucial in various fields, from engineering and physics to chemistry and environmental science. Two fundamental processes often causing confusion are isothermal and adiabatic processes. While both involve changes in a system's state, they differ significantly in how heat and work interact. This comprehensive article delves into the core differences between isothermal and adiabatic processes, exploring their defining characteristics, practical applications, and relevant equations.
Defining Isothermal Processes
An isothermal process is a thermodynamic process that occurs at a constant temperature. This implies that the system remains in thermal equilibrium with its surroundings throughout the entire process. Heat transfer can occur freely between the system and its environment, ensuring the temperature remains unchanged. This constant temperature is maintained through slow, gradual changes or by placing the system in a large thermal reservoir. The hallmark of an isothermal process is that ΔT = 0 (change in temperature is zero).
Key Characteristics of Isothermal Processes:
- Constant Temperature: The most defining characteristic; temperature remains unchanged throughout the process.
- Heat Transfer: Heat exchange with the surroundings is possible and often necessary to maintain the constant temperature.
- Slow Processes: Isothermal processes typically occur slowly to allow ample time for heat transfer and maintain thermal equilibrium.
- Work Done: Work can be done on or by the system, leading to changes in internal energy and volume.
- Ideal Gas Law Applicability: The ideal gas law (PV = nRT) is directly applicable to isothermal processes involving ideal gases.
Examples of Isothermal Processes:
- Melting of Ice: The phase transition of ice into water at 0°C is an isothermal process, assuming sufficient heat exchange with the surroundings.
- Boiling of Water: Similarly, the boiling of water at 100°C (at standard atmospheric pressure) is isothermal, provided heat transfer is allowed to maintain the temperature.
- Expansion of a Gas in a Thermal Bath: A gas expanding slowly in a large thermal bath will maintain a constant temperature throughout the expansion.
- Many biological processes: Many biological reactions occur under isothermal conditions, due to the temperature regulating mechanisms in living organisms.
Defining Adiabatic Processes
In contrast to isothermal processes, an adiabatic process is one that occurs without any heat transfer into or out of the system. This means that the system is perfectly insulated from its surroundings. Consequently, the temperature of the system may change during an adiabatic process, depending on whether work is done on or by the system.
Key Characteristics of Adiabatic Processes:
- No Heat Transfer: The defining feature; there is no heat exchange (Q = 0) between the system and its surroundings.
- Temperature Change: The temperature of the system can change significantly during an adiabatic process.
- Fast Processes: Adiabatic processes typically occur rapidly, preventing significant heat transfer.
- Work Done: Work can be done on or by the system, directly affecting the internal energy and temperature.
- Adiabatic Equation Applicability: The adiabatic equation (PV<sup>γ</sup> = constant) governs the relationship between pressure and volume during an adiabatic process, where γ is the adiabatic index (ratio of specific heats).
Examples of Adiabatic Processes:
- Rapid Expansion or Compression of a Gas: The sudden expansion or compression of a gas, such as in a piston cylinder system, occurs adiabatically if the process is fast enough to prevent significant heat transfer.
- Cloud Formation: The adiabatic cooling of rising air masses in the atmosphere leads to cloud formation as the water vapor condenses.
- Internal Combustion Engine: The power stroke in an internal combustion engine is approximately an adiabatic process, due to the rapid combustion and expansion of gases.
- Certain Meteorological Phenomena: Adiabatic processes play a vital role in various meteorological phenomena like the formation of storms and the movement of air masses.
Comparing Isothermal and Adiabatic Processes: A Table Summary
Feature | Isothermal Process | Adiabatic Process |
---|---|---|
Heat Transfer | Q ≠ 0 (heat transfer occurs) | Q = 0 (no heat transfer) |
Temperature | Constant temperature (ΔT = 0) | Temperature may change (ΔT ≠ 0) |
Process Speed | Typically slow | Typically fast |
Governing Law | Ideal Gas Law (PV = nRT) for ideal gases | Adiabatic Equation (PV<sup>γ</sup> = constant) for ideal gases |
Work Done | Work can be done, affecting internal energy | Work can be done, directly affecting temperature |
System Isolation | System is in thermal contact with surroundings | System is thermally isolated from surroundings |
Equations Governing Isothermal and Adiabatic Processes
The behaviour of ideal gases under isothermal and adiabatic conditions is described by specific equations:
Isothermal Process:
For an ideal gas undergoing an isothermal process, the ideal gas law applies:
PV = nRT
where:
- P = pressure
- V = volume
- n = number of moles
- R = ideal gas constant
- T = temperature (constant)
This equation shows the inverse relationship between pressure and volume at a constant temperature.
Adiabatic Process:
For an ideal gas undergoing an adiabatic process, the adiabatic equation applies:
PV<sup>γ</sup> = constant
where:
- P = pressure
- V = volume
- γ = adiabatic index (ratio of specific heats, C<sub>p</sub>/C<sub>v</sub>)
The adiabatic index, γ, represents the ratio of the specific heat at constant pressure (C<sub>p</sub>) to the specific heat at constant volume (C<sub>v</sub>). Its value depends on the nature of the gas. For a monatomic ideal gas, γ = 5/3; for a diatomic ideal gas, γ = 7/5.
Practical Applications and Implications
Understanding the differences between isothermal and adiabatic processes is essential in various fields:
Engineering:
- Internal Combustion Engines: While not perfectly adiabatic, the power stroke in an internal combustion engine approximates an adiabatic process, affecting the engine's efficiency and power output.
- Refrigeration and Air Conditioning: Understanding adiabatic expansion and compression is crucial in designing efficient refrigeration and air conditioning systems.
- Gas Turbines: Adiabatic processes are central to the functioning of gas turbines, determining their performance and efficiency.
- Chemical Processes: Many industrial chemical processes involve carefully controlled isothermal or adiabatic conditions to optimize reactions and yields.
Meteorology and Climatology:
- Cloud Formation: Adiabatic cooling of air masses is a primary factor in cloud formation and precipitation.
- Atmospheric Dynamics: Adiabatic processes play a significant role in various atmospheric phenomena, including the formation of storms and the movement of air masses.
Biological Systems:
- Metabolic Processes: Many biological processes strive to maintain near-isothermal conditions, minimizing temperature fluctuations and ensuring optimal enzymatic activity.
Conclusion
Isothermal and adiabatic processes represent two fundamental types of thermodynamic processes with distinct characteristics. Isothermal processes maintain constant temperature through heat exchange, while adiabatic processes occur without heat transfer, allowing temperature changes due to work done. Understanding these differences is crucial for analyzing and predicting the behavior of various systems in diverse fields. While ideal conditions of perfect insulation or unrestricted heat flow are rarely achieved in practice, these models provide valuable approximations that enable us to understand and design many real-world systems and processes. By grasping the core principles and applying the relevant equations, engineers, scientists, and researchers can accurately model and predict the behavior of systems undergoing isothermal and adiabatic changes.
Latest Posts
Latest Posts
-
Carbohydrates Are Made Of What Elements
May 10, 2025
-
Identify Whether The Following Compound Is Aromatic
May 10, 2025
-
Which Option Is A Physical Property Of Matter
May 10, 2025
-
What Are Properties Of A Compound
May 10, 2025
-
Covalent Chemical Bond Is One In Which
May 10, 2025
Related Post
Thank you for visiting our website which covers about Difference Between Isothermal And Adiabatic Process . We hope the information provided has been useful to you. Feel free to contact us if you have any questions or need further assistance. See you next time and don't miss to bookmark.