Differential Scanning Calorimetry Glass Transition Temperature
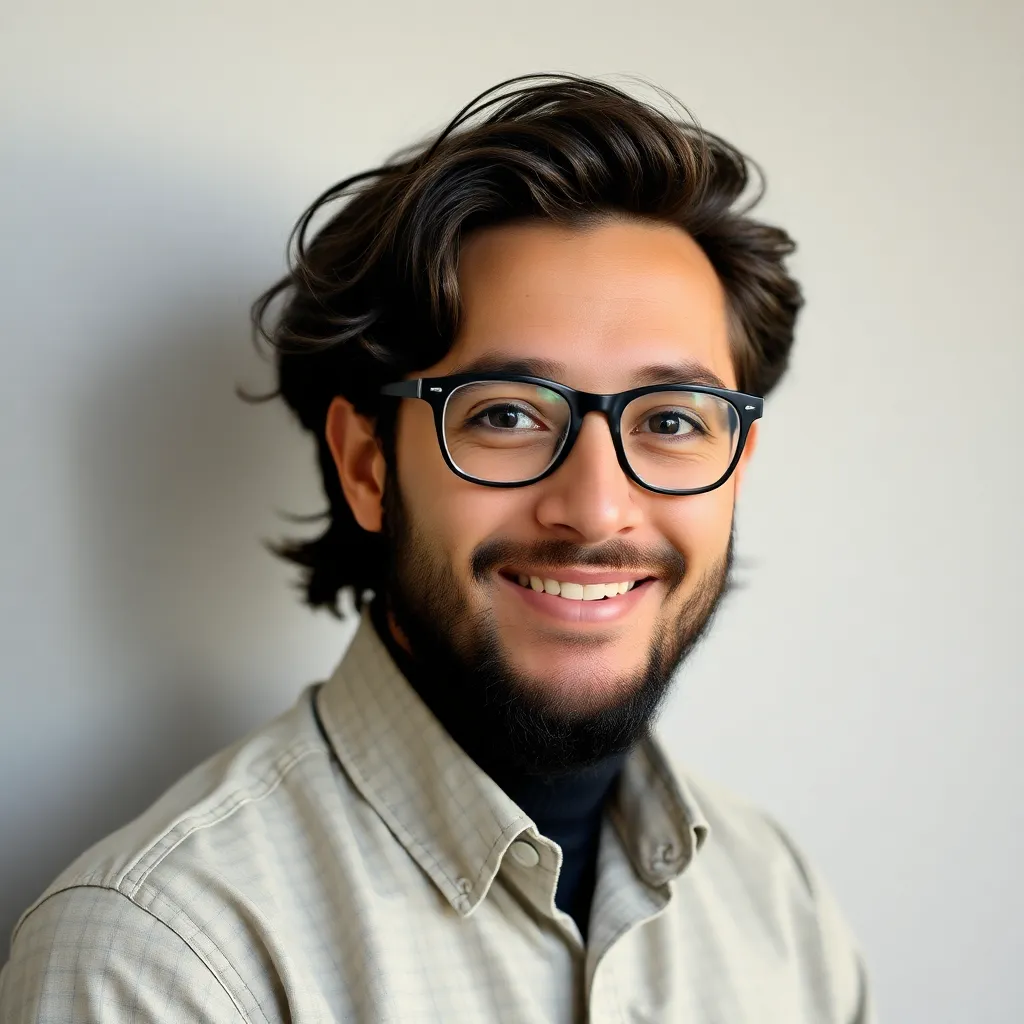
Muz Play
Mar 30, 2025 · 7 min read
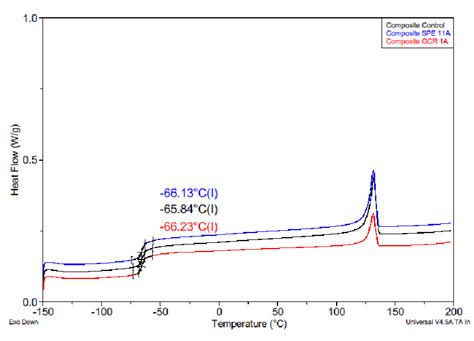
Table of Contents
Differential Scanning Calorimetry (DSC) and Glass Transition Temperature (Tg)
Differential Scanning Calorimetry (DSC) is a thermoanalytic technique widely used to study the thermal properties of materials. It measures the difference in heat flow between a sample and a reference as a function of temperature or time. One of its most important applications is determining the glass transition temperature (Tg), a crucial characteristic of amorphous materials, including polymers, glasses, and pharmaceuticals. Understanding Tg is vital for predicting material behavior and performance across various applications. This comprehensive article will delve into the principles of DSC, its application in Tg determination, factors influencing Tg measurements, and the significance of Tg in material science.
Understanding Glass Transition
Before exploring DSC's role, let's clarify the concept of the glass transition. Unlike crystalline materials that exhibit a sharp melting point, amorphous materials undergo a gradual transition from a hard, glassy state to a more rubbery or viscous state. This transition isn't a true thermodynamic phase change like melting; it's a change in the material's dynamic properties. At the glass transition temperature (Tg), the material's viscosity increases dramatically, leading to changes in its mechanical, thermal, and electrical properties.
Molecular Mobility and Tg
At temperatures below Tg, the molecules in an amorphous material are essentially frozen in place, exhibiting minimal molecular mobility. As the temperature increases towards Tg, molecular motion begins to increase gradually. At Tg, the molecules gain sufficient kinetic energy to overcome intermolecular forces, resulting in significant segmental motion and an increase in free volume. This increased mobility is responsible for the observed changes in the material's physical properties.
Differential Scanning Calorimetry (DSC): The Technique
DSC measures the heat flow difference between a sample and an inert reference as they are subjected to a controlled temperature program. This difference in heat flow, expressed as heat flow rate (mW or J/s), reveals information about the material's thermal transitions, including the glass transition.
Types of DSC: Modulated and Conventional
There are two main types of DSC: conventional (or power-compensated) and modulated (MDSC). Conventional DSC measures the total heat flow, including both reversible and irreversible processes. MDSC, on the other hand, superimposes a sinusoidal temperature modulation onto a linear temperature ramp. This allows for the separation of heat flow contributions from different processes, providing a more detailed analysis of complex thermal events. While both can determine Tg, MDSC offers superior resolution and the ability to separate overlapping transitions, especially beneficial in complex materials.
The DSC Curve and Tg Identification
A typical DSC curve plots heat flow against temperature. At the glass transition, a step change or inflection in the baseline is observed. This change is not a sharp peak like melting or crystallization; instead, it's a gradual shift in the baseline. The Tg is determined by drawing a baseline tangent to the curve before and after the transition, and the midpoint of the transition is taken as Tg. Different software packages might use slightly varying methods to determine the midpoint, potentially leading to small variations in the reported Tg value. Precise baseline construction is crucial for accurate Tg determination.
Factors Influencing Tg Measurement by DSC
Several factors can influence the measured Tg value obtained through DSC, affecting the accuracy and reproducibility of the results. These include:
1. Heating Rate:
The heating rate significantly impacts Tg. Faster heating rates typically result in higher Tg values because the molecules don't have enough time to relax and achieve equilibrium. Slower heating rates, conversely, generally lead to lower Tg values. A standardized heating rate is crucial for consistency and comparability between measurements.
2. Sample Mass:
The sample mass also plays a role. Too large a sample might lead to temperature gradients within the sample pan, resulting in inaccurate Tg determination. A small, evenly distributed sample is recommended.
3. Thermal History:
The thermal history of the sample heavily influences Tg. A sample that has been subjected to different thermal treatments (e.g., annealing, quenching) will have a different Tg. Prior thermal treatments can impact the molecular arrangement and free volume of the material. This highlights the importance of standardizing sample preparation to ensure reliable data.
4. Instrument Calibration:
Accurate instrument calibration is essential. The DSC instrument must be properly calibrated using certified standards to ensure accurate temperature and heat flow measurements. This is paramount for ensuring the precision and accuracy of Tg determination.
5. Atmosphere:
The atmosphere (inert gas like nitrogen or air) surrounding the sample can influence Tg. The presence of oxygen or moisture might affect the material's properties, consequently influencing the transition temperature. A controlled atmosphere is therefore necessary for consistent results.
6. Sample Preparation:
Careful sample preparation is crucial. The sample must be homogenous and free of any contaminants or moisture. Properly prepared samples minimize experimental errors and ensure the reliability of Tg measurements. Variations in sample preparation techniques can affect the apparent Tg value, emphasizing the need for consistent protocols.
Applications of Tg Determination by DSC
The determination of Tg using DSC has numerous applications across diverse scientific and industrial fields. Its importance stems from the strong correlation between Tg and a material's properties:
1. Polymer Science:
Tg is a key parameter in polymer science, directly impacting the material's mechanical properties, such as stiffness, flexibility, and impact resistance. It defines the temperature range over which a polymer transitions from a hard, brittle state to a more flexible, rubbery state. This allows engineers to select polymers appropriate for a specific application based on the operating temperature range.
2. Pharmaceutical Industry:
In pharmaceuticals, Tg is crucial for understanding the physical stability and processability of drugs and drug formulations. Tg values aid in predicting the shelf life of solid dosage forms and guiding the development of optimal formulations. A drug's Tg value can help determine the formulation's stability and potential for phase separation or crystallization, impacting its efficacy and safety.
3. Food Science:
Tg is also relevant in food science, affecting the texture and shelf life of various food products. Understanding the Tg of food components can inform processing techniques and predict changes in food texture during storage.
4. Glass Technology:
The Tg of glasses directly influences their properties, especially their viscosity and ability to undergo shaping processes. Tg determination by DSC is vital for controlling glass manufacturing processes.
5. Materials Science Research:
DSC analysis is extensively used in materials science research to study the glass transition behavior of novel materials. The Tg data helps in understanding the molecular dynamics and structure-property relationships of amorphous materials.
Advanced Techniques and Data Analysis
While conventional DSC is widely used, advanced techniques and data analysis methods are employed to enhance the accuracy and information derived from DSC measurements.
1. Modulated DSC (MDSC):
As mentioned previously, MDSC separates reversible and non-reversible heat flow contributions, offering a more detailed understanding of the glass transition. This allows for the identification of subtle transitions that might be masked in conventional DSC.
2. Step-Change DSC:
Step-change DSC involves inducing isothermal steps within the temperature ramp. This allows for a more detailed analysis of the kinetics of the glass transition.
3. Kissinger Method:
This method utilizes DSC data from multiple heating rates to determine the activation energy associated with the glass transition.
4. Curve Fitting and Modeling:
Sophisticated curve-fitting techniques and modeling can be used to extract additional information from DSC data, including the relaxation time of the polymer chains and the activation energy of the glass transition.
Conclusion
Differential Scanning Calorimetry is a powerful technique for determining the glass transition temperature (Tg) of amorphous materials. The Tg value, a critical material property, influences many aspects of material behavior and performance. Understanding the principles of DSC, factors influencing Tg measurements, and the applications of Tg determination across various fields is essential for materials scientists, engineers, and researchers. While standard DSC is widely used, advanced techniques like MDSC and sophisticated data analysis methods provide even more detailed insights into the complex phenomenon of the glass transition. The continued development and refinement of DSC techniques will undoubtedly further enhance our ability to characterize and understand the behavior of amorphous materials.
Latest Posts
Latest Posts
-
What Is The Difference Between Cellular Respiration And Fermentation
Apr 01, 2025
-
What Are Two Functional Groups Found In Amino Acids
Apr 01, 2025
-
Foundations Of Education 13th Edition Pdf Free Download
Apr 01, 2025
-
Does Lead Need A Roman Numeral
Apr 01, 2025
-
Introduction To Chemical Reactions Answer Key
Apr 01, 2025
Related Post
Thank you for visiting our website which covers about Differential Scanning Calorimetry Glass Transition Temperature . We hope the information provided has been useful to you. Feel free to contact us if you have any questions or need further assistance. See you next time and don't miss to bookmark.