Dna And Protein Synthesis Study Guide
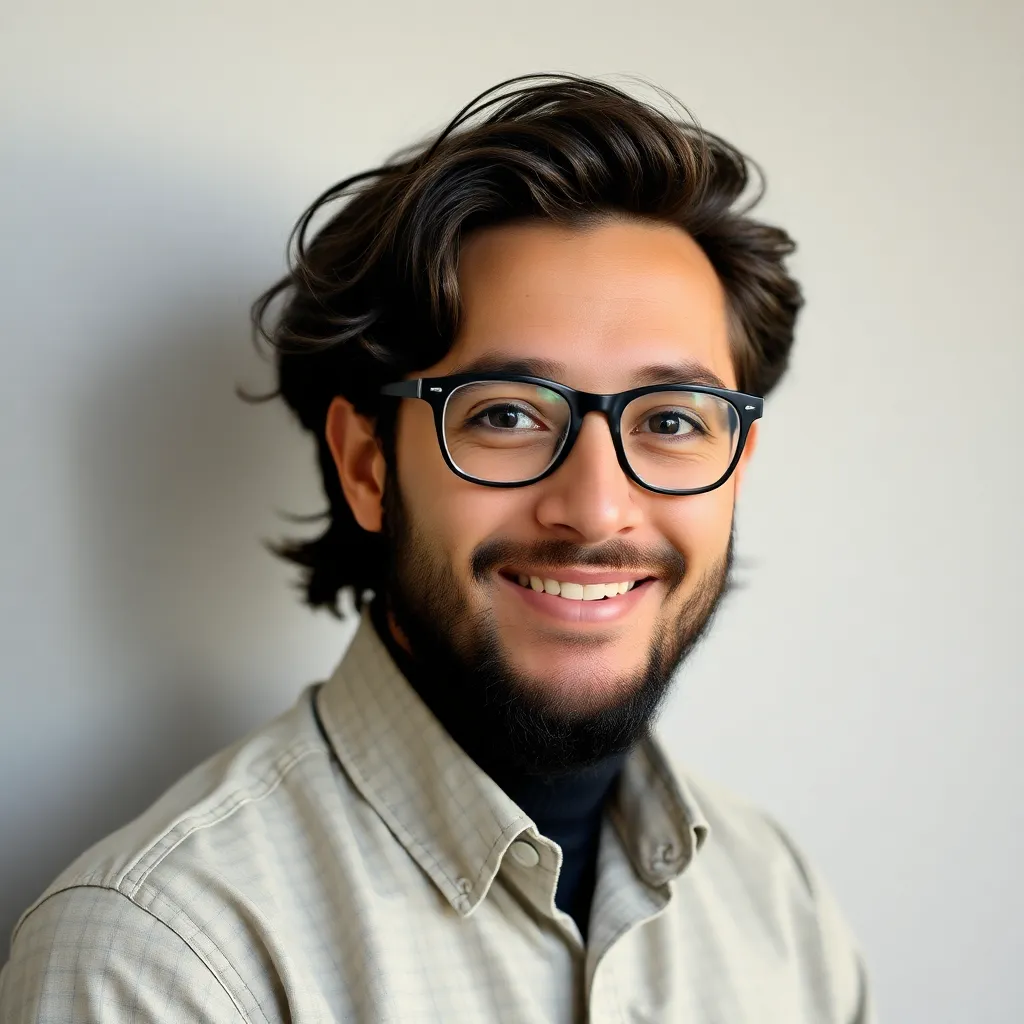
Muz Play
May 10, 2025 · 8 min read
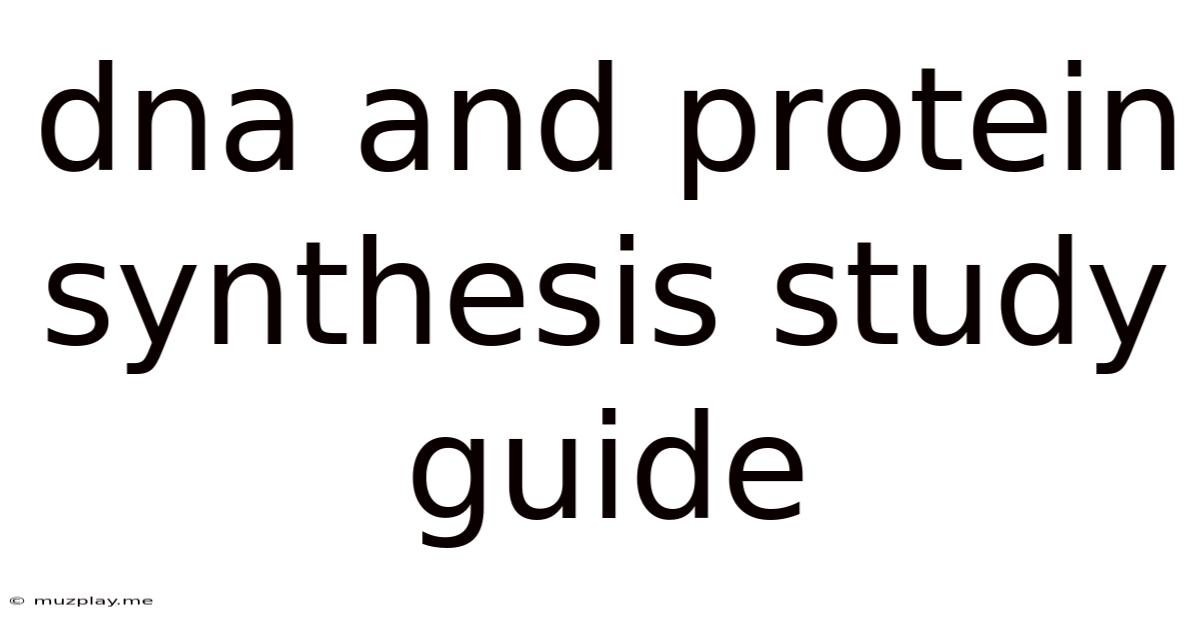
Table of Contents
DNA and Protein Synthesis Study Guide: A Comprehensive Overview
This comprehensive study guide delves into the fascinating world of DNA and protein synthesis. Understanding these processes is crucial for grasping fundamental concepts in biology and related fields. We'll explore DNA structure, replication, transcription, translation, and the regulation of these processes, providing you with a solid foundation for your studies.
I. DNA: The Blueprint of Life
Deoxyribonucleic acid (DNA) is the hereditary material in humans and almost all other organisms. It's a double-stranded helix composed of nucleotides, each containing a deoxyribose sugar, a phosphate group, and one of four nitrogenous bases: adenine (A), guanine (G), cytosine (C), and thymine (T). The structure, famously discovered by Watson and Crick, is crucial to its function.
A. DNA Structure: The Double Helix
The double helix structure is characterized by two complementary strands running antiparallel to each other (one 5' to 3', the other 3' to 5'). The bases pair specifically: adenine always pairs with thymine (A-T) via two hydrogen bonds, and guanine always pairs with cytosine (G-C) via three hydrogen bonds. This specific base pairing is fundamental to DNA replication and transcription. The sugar-phosphate backbone forms the outside of the helix, while the bases are stacked in the interior.
B. DNA Replication: Making Copies
DNA replication is the process of creating an identical copy of a DNA molecule. It's a semiconservative process, meaning each new DNA molecule consists of one original strand and one newly synthesized strand. Several key enzymes are involved:
- Helicase: Unwinds the DNA double helix.
- Primase: Synthesizes RNA primers, providing a starting point for DNA polymerase.
- DNA Polymerase: Adds nucleotides to the growing DNA strand, always in the 5' to 3' direction. It also proofreads the newly synthesized strand for errors.
- Ligase: Joins Okazaki fragments (short DNA fragments synthesized on the lagging strand) together.
The replication process occurs in several steps: initiation, elongation, and termination. Understanding the roles of each enzyme and the directionality of DNA synthesis is critical. The leading strand is synthesized continuously, while the lagging strand is synthesized discontinuously in short fragments.
II. From DNA to Protein: The Central Dogma
The central dogma of molecular biology describes the flow of genetic information: DNA → RNA → Protein. This involves two main processes: transcription and translation.
III. Transcription: DNA to RNA
Transcription is the process of synthesizing RNA from a DNA template. The enzyme RNA polymerase is responsible for this process. Unlike DNA replication, transcription only involves one strand of the DNA molecule, the template strand. The RNA molecule synthesized is complementary to the template strand and identical to the coding strand (except uracil (U) replaces thymine (T)).
A. Types of RNA
Several types of RNA are involved in protein synthesis:
- Messenger RNA (mRNA): Carries the genetic code from DNA to the ribosomes.
- Transfer RNA (tRNA): Carries amino acids to the ribosomes during translation.
- Ribosomal RNA (rRNA): Forms part of the ribosome structure.
B. Transcription Stages
Transcription involves three main stages:
- Initiation: RNA polymerase binds to a promoter region on the DNA molecule.
- Elongation: RNA polymerase synthesizes the RNA molecule, adding nucleotides in the 5' to 3' direction.
- Termination: RNA polymerase reaches a terminator sequence on the DNA molecule and releases the RNA molecule.
Post-transcriptional modifications, such as splicing (removal of introns and joining of exons) and capping and tailing (addition of a 5' cap and a poly-A tail), are crucial for mRNA stability and translation efficiency in eukaryotes. Prokaryotes, however, lack these modifications.
IV. Translation: RNA to Protein
Translation is the process of synthesizing a protein from an mRNA template. This occurs in the ribosomes, which are complex molecular machines composed of rRNA and proteins.
A. The Genetic Code
The genetic code is a set of rules that specifies the correspondence between mRNA codons (three-nucleotide sequences) and amino acids. There are 64 possible codons, with each codon specifying either an amino acid or a stop signal. The code is degenerate, meaning multiple codons can specify the same amino acid. This redundancy helps protect against mutations.
B. tRNA and Aminoacyl-tRNA Synthetases
tRNA molecules are adapter molecules that carry amino acids to the ribosomes. Each tRNA molecule has an anticodon that is complementary to a specific mRNA codon. Aminoacyl-tRNA synthetases are enzymes that attach the correct amino acid to its corresponding tRNA molecule. This ensures the accurate translation of the genetic code.
C. Ribosomes and Translation Stages
Ribosomes have three binding sites for tRNA molecules: A (aminoacyl), P (peptidyl), and E (exit). Translation proceeds in three stages:
- Initiation: The ribosome binds to the mRNA molecule and the initiator tRNA (carrying methionine).
- Elongation: The ribosome moves along the mRNA molecule, adding amino acids to the growing polypeptide chain. This involves codon recognition, peptide bond formation, and translocation.
- Termination: The ribosome reaches a stop codon, and the polypeptide chain is released.
After translation, the polypeptide chain folds into a specific three-dimensional structure, often requiring chaperone proteins to assist in the process. Post-translational modifications, such as glycosylation and phosphorylation, can further alter the protein's structure and function.
V. Regulation of Gene Expression
Gene expression is the process by which the information encoded in a gene is used to synthesize a functional gene product, typically a protein. The regulation of gene expression is crucial for controlling cellular processes and adapting to environmental changes.
A. Prokaryotic Regulation: The Operon Model
Prokaryotes, such as bacteria, often regulate gene expression using operons. An operon is a cluster of genes that are transcribed together and regulated by a single promoter. The lac operon is a classic example, controlling the expression of genes involved in lactose metabolism. Its regulation involves repressors and activators that bind to specific DNA sequences, affecting RNA polymerase binding and transcription initiation.
B. Eukaryotic Regulation: A Multi-Layered Approach
Eukaryotic gene regulation is much more complex than prokaryotic regulation. It involves multiple levels of control, including:
- Chromatin remodeling: Changes in the structure of chromatin (DNA and associated proteins) can affect the accessibility of genes to RNA polymerase.
- Transcriptional regulation: Transcription factors bind to specific DNA sequences, either activating or repressing transcription.
- RNA processing: Post-transcriptional modifications, such as splicing and alternative splicing, can affect the production of different protein isoforms from a single gene.
- RNA stability and degradation: The lifespan of mRNA molecules can be regulated, affecting the amount of protein produced.
- Translational regulation: The rate of translation can be controlled by factors affecting ribosome binding and initiation.
- Post-translational modification: Modifications to the protein after translation can affect its activity and lifespan.
These multiple levels of regulation provide precise control over gene expression, ensuring that proteins are produced only when and where they are needed.
VI. Mutations and their Effects
Mutations are changes in the DNA sequence. They can be caused by various factors, including errors during DNA replication, exposure to mutagens (e.g., radiation, chemicals), or transposable elements (jumping genes). Mutations can have a wide range of effects, from no noticeable effect to significant changes in phenotype (observable characteristics).
A. Types of Mutations
Mutations can be classified into several types:
- Point mutations: Changes in a single nucleotide. These can be substitutions (one base replaced by another), insertions, or deletions. Substitutions can be missense (changing one amino acid), nonsense (creating a stop codon), or silent (no change in amino acid sequence).
- Frameshift mutations: Insertions or deletions that are not multiples of three nucleotides. These shift the reading frame of the mRNA molecule, resulting in a completely different amino acid sequence downstream of the mutation.
- Chromosomal mutations: Larger-scale changes affecting entire chromosomes or large segments of chromosomes. These include deletions, duplications, inversions, and translocations.
B. Effects of Mutations
The effects of mutations depend on several factors, including:
- The type of mutation: Some mutations have a more significant impact than others.
- The location of the mutation: Mutations in coding regions generally have a greater effect than mutations in non-coding regions.
- The function of the affected gene: Mutations in genes with crucial functions can have severe consequences.
Many mutations are harmful, causing genetic disorders or diseases. However, some mutations can be beneficial, providing the raw material for evolution. Understanding the types and effects of mutations is crucial for understanding genetic diseases and evolution.
VII. Techniques Used to Study DNA and Protein Synthesis
Several techniques are used to study DNA and protein synthesis:
- Polymerase chain reaction (PCR): Amplifies specific DNA sequences.
- Gel electrophoresis: Separates DNA or proteins based on size and charge.
- DNA sequencing: Determines the exact order of nucleotides in a DNA molecule.
- Gene cloning: Creates multiple copies of a specific gene.
- Gene editing: Modifies the DNA sequence using tools like CRISPR-Cas9.
- Protein purification and analysis: Isolates and characterizes proteins.
- Immunoblotting (Western blotting): Detects specific proteins using antibodies.
These techniques provide powerful tools for investigating the processes of DNA replication, transcription, translation, and the regulation of gene expression.
This comprehensive study guide provides a strong foundation for understanding DNA and protein synthesis. Remember to utilize additional resources, practice problems, and seek clarification when needed to fully grasp these crucial biological processes. Good luck with your studies!
Latest Posts
Latest Posts
-
The Malonic Ester Synthesis Is A Method For Preparing
May 10, 2025
-
Write The Electron Configuration For A Neutral Atom Of Sodium
May 10, 2025
-
Which Process Squeezes Layers Of Sediment Together
May 10, 2025
-
What Is The Periodic Trend For First Ionization Energy
May 10, 2025
-
Sociologists Using Conflict Perspective Might Study What
May 10, 2025
Related Post
Thank you for visiting our website which covers about Dna And Protein Synthesis Study Guide . We hope the information provided has been useful to you. Feel free to contact us if you have any questions or need further assistance. See you next time and don't miss to bookmark.