During Electrolysis Of H2so4 With High Charge Density
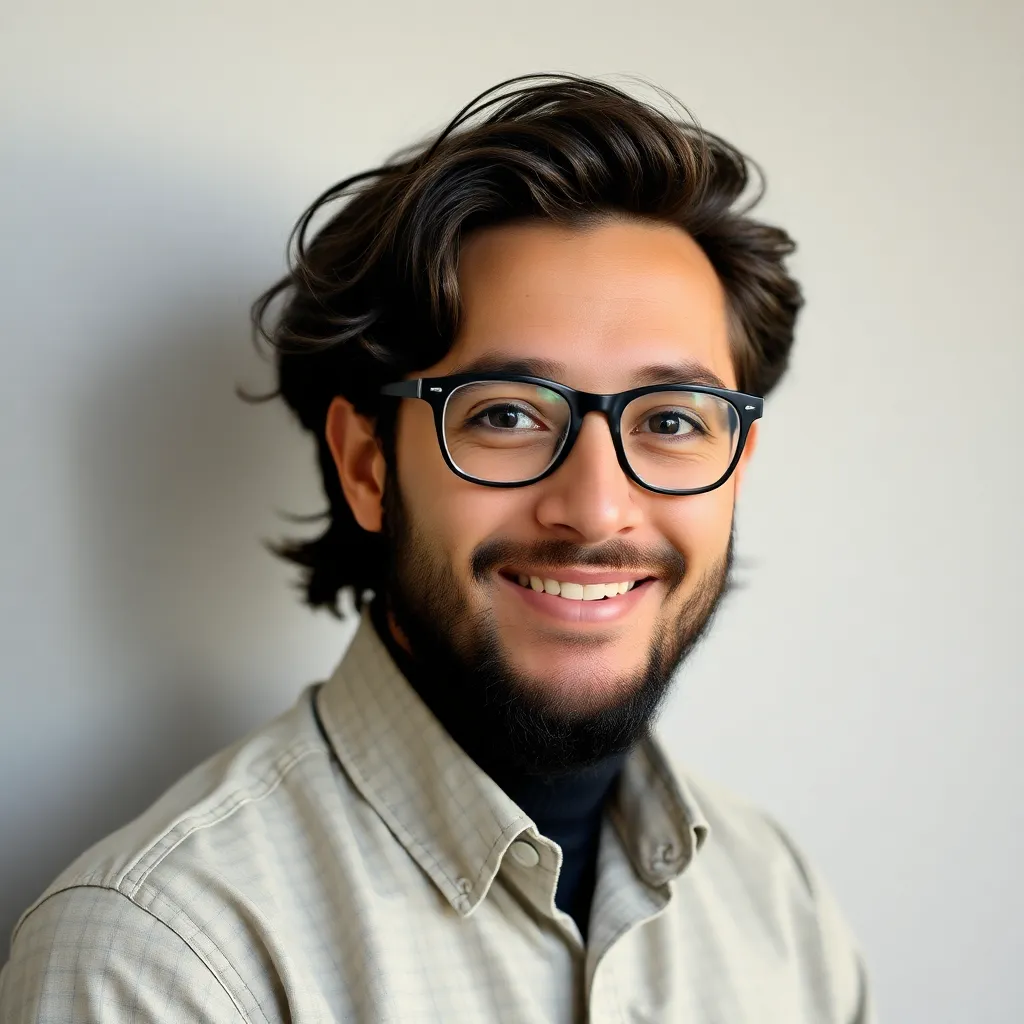
Muz Play
Apr 25, 2025 · 6 min read

Table of Contents
During Electrolysis of H₂SO₄ with High Charge Density: A Deep Dive into Electrochemical Phenomena
Electrolysis, the process of using electricity to drive non-spontaneous chemical reactions, presents fascinating complexities when applied to strong electrolytes like sulfuric acid (H₂SO₄) under conditions of high charge density. This scenario significantly alters the electrochemical landscape, impacting reaction pathways, product formation, and overall efficiency. This article explores the intricate processes involved during the electrolysis of H₂SO₄ at high charge density, examining the influence of various factors and highlighting the practical implications.
Understanding the Basics of H₂SO₄ Electrolysis
Before delving into high charge density scenarios, let's establish a foundational understanding of H₂SO₄ electrolysis at lower current densities. In aqueous solutions, sulfuric acid dissociates completely into hydrogen ions (H⁺) and sulfate ions (SO₄²⁻). During electrolysis, these ions migrate towards their respective electrodes:
-
At the Cathode (Negative Electrode): Hydrogen ions (H⁺) undergo reduction, gaining electrons to form hydrogen gas (H₂). The reaction is: 2H⁺ + 2e⁻ → H₂
-
At the Anode (Positive Electrode): The primary reaction involves the oxidation of water molecules (H₂O) to produce oxygen gas (O₂) and hydrogen ions (H⁺). This is because the oxidation of sulfate ions is kinetically hindered. The reaction is: 2H₂O → O₂ + 4H⁺ + 4e⁻
Important Note: While the oxidation of sulfate is thermodynamically favorable, the high overpotential required makes water oxidation the dominant process at the anode in most scenarios, unless highly specific electrode materials are used.
The Impact of High Charge Density
Increasing the charge density (current density) significantly alters the dynamics of this electrolytic process. Several key effects come into play:
1. Enhanced Reaction Rates:
Higher charge densities translate to a greater flux of electrons at the electrodes, accelerating both the reduction at the cathode and the oxidation at the anode. This leads to a more rapid production of hydrogen and oxygen gases. However, this increase isn't linear; there are limitations imposed by mass transport and electrochemical kinetics.
2. Overpotential Effects:
Overpotential, the extra voltage required beyond the thermodynamic potential to drive a reaction at a specific rate, becomes increasingly significant at high charge densities. This is because the rate of electron transfer and mass transport to the electrode surface become limiting factors. The increased overpotential can lead to:
- Increased energy consumption: More energy is required to achieve the same level of gas production.
- Side reactions: Higher overpotentials can favor less favorable electrochemical reactions, potentially leading to the formation of unwanted byproducts. This could include the oxidation of sulfate ions at the anode under extreme conditions, though it remains unlikely without specialized electrode materials.
3. Mass Transport Limitations:
At high charge densities, the rate of reactant consumption at the electrode surface can outpace the rate at which fresh reactants are supplied through diffusion or convection. This creates concentration gradients near the electrode, leading to:
- Depletion of reactants: The concentration of H⁺ ions near the cathode and H₂O molecules near the anode can decrease significantly, slowing down the main reactions.
- Formation of concentration polarization: The difference in concentration between the bulk solution and the electrode surface creates a potential difference, further influencing the overall cell potential and reaction pathways.
4. Temperature Effects:
Joule heating, caused by the passage of high current through the electrolyte, leads to a significant increase in temperature. This temperature rise can have several implications:
- Increased reaction rates: Higher temperatures generally accelerate reaction kinetics, potentially offsetting some of the negative impacts of mass transport limitations.
- Changes in electrolyte properties: The conductivity and viscosity of the H₂SO₄ solution change with temperature, impacting ion mobility and mass transport.
- Gas solubility: The solubility of hydrogen and oxygen gases in the solution decreases with increasing temperature, potentially leading to bubble formation and changes in the electrode-electrolyte interface.
5. Electrode Material Effects:
The choice of electrode material is crucial, especially under high charge density conditions. Different materials exhibit varying overpotentials for hydrogen and oxygen evolution, influencing the efficiency and selectivity of the process. Electrode degradation due to corrosion or passivation can also become more prominent at high current densities.
Analyzing the Faradaic Efficiency
Faradaic efficiency, a crucial parameter in electrolysis, represents the ratio of the actual amount of product obtained to the theoretical amount expected based on Faraday's law. High charge density can negatively impact Faradaic efficiency due to the aforementioned factors:
- Side reactions: The occurrence of unwanted reactions consumes charge without contributing to the desired hydrogen and oxygen production.
- Mass transport limitations: Incomplete conversion of reactants due to concentration polarization reduces the overall yield.
Maintaining high Faradaic efficiency under high charge density conditions requires careful control of various parameters, including current density, temperature, electrolyte concentration, and electrode material.
Practical Implications and Applications
The electrolysis of H₂SO₄ at high charge density, despite the challenges, finds application in several areas:
- Hydrogen Production: While there are more efficient ways to produce hydrogen, high charge density electrolysis can be employed in niche applications requiring localized, high-rate hydrogen generation.
- Oxygen Production: Similarly, high-purity oxygen production can be achieved through this method, although other technologies are generally preferred for large-scale oxygen generation.
- Electrochemical Synthesis: Under controlled conditions and with specific electrode materials, high charge density electrolysis of H₂SO₄ can be used in the synthesis of certain compounds. This is often less efficient than other methods.
- Electrochemical Machining: While not directly related to the generation of hydrogen and oxygen, the high current densities used can be employed in electrochemical machining processes.
Future Research Directions
Further research into high charge density electrolysis of H₂SO₄ is essential for improving its efficiency and expanding its applications. Key areas include:
- Development of advanced electrode materials: Research into new materials with improved catalytic activity and resistance to corrosion is crucial for enhancing the process's efficiency and longevity.
- Optimization of cell design: Innovative cell designs that enhance mass transport and minimize concentration polarization are vital for achieving higher current densities without compromising Faradaic efficiency.
- Computational modeling: Advanced computational models can provide insights into the complex electrochemical processes occurring at high charge densities, enabling better process optimization.
- Exploring alternative electrolytes: Investigating alternative electrolytes with improved properties at high current densities could lead to enhanced performance.
Conclusion
Electrolysis of sulfuric acid at high charge density presents a complex interplay of electrochemical phenomena. While high current densities accelerate gas production, they also introduce challenges related to overpotential, mass transport limitations, temperature effects, and Faradaic efficiency. Understanding these complexities and implementing suitable mitigation strategies is essential for harnessing the potential of this process in various applications. Ongoing research focused on advanced materials, optimized cell design, and sophisticated modeling techniques will be crucial in unlocking the full potential of high charge density electrolysis of H₂SO₄. The potential for improved efficiency and broader applications makes this a vibrant area of ongoing investigation within electrochemistry.
Latest Posts
Latest Posts
-
Do Saturated Or Unsaturated Fats Have A Higher Melting Point
Apr 26, 2025
-
For Each Alcohol Reaction Give The Major Product
Apr 26, 2025
-
Give The Complete Electron Configuration For Mn
Apr 26, 2025
-
Correctly Label The Cross Section Of The Thigh
Apr 26, 2025
-
All The Elements In Group 2 Have The Same
Apr 26, 2025
Related Post
Thank you for visiting our website which covers about During Electrolysis Of H2so4 With High Charge Density . We hope the information provided has been useful to you. Feel free to contact us if you have any questions or need further assistance. See you next time and don't miss to bookmark.