During The Electron Transport Chain Atp Will Be Made From
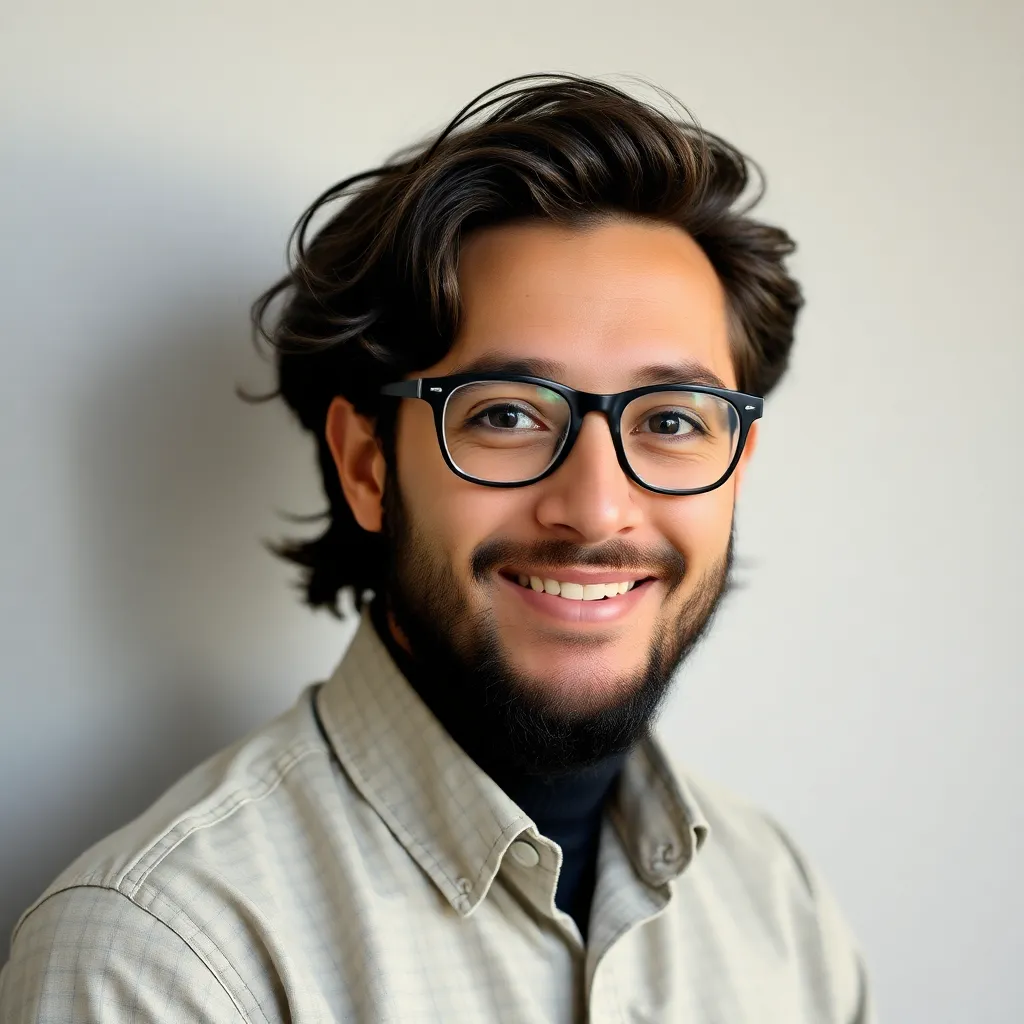
Muz Play
May 11, 2025 · 6 min read
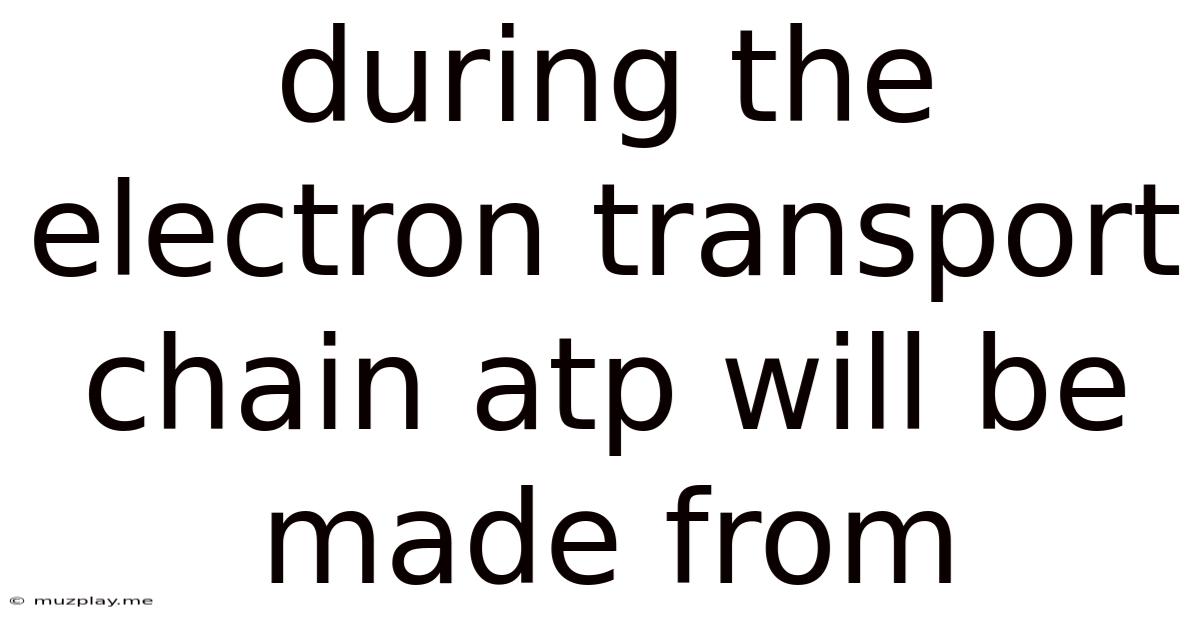
Table of Contents
During the Electron Transport Chain, ATP Will Be Made From: A Deep Dive into Oxidative Phosphorylation
The electron transport chain (ETC), also known as the respiratory chain, is the final stage of cellular respiration, a crucial process that generates the majority of adenosine triphosphate (ATP), the cell's primary energy currency. Understanding how ATP is made during the ETC is key to comprehending cellular energy metabolism. This process, known as oxidative phosphorylation, is remarkably efficient, extracting significant energy from electrons harvested earlier in cellular respiration. This article will delve into the intricate mechanisms of oxidative phosphorylation, exploring the precise steps and components involved in ATP synthesis during the ETC.
The Precursors: NADH and FADH2
Before embarking on the journey through the ETC, it's crucial to understand the role of nicotinamide adenine dinucleotide (NADH) and flavin adenine dinucleotide (FADH2). These two electron carriers are generated during the preceding stages of cellular respiration – glycolysis and the Krebs cycle (also known as the citric acid cycle). They act as crucial links, transporting high-energy electrons to the ETC.
-
NADH: Produced during glycolysis and the Krebs cycle, NADH carries a pair of high-energy electrons. Its role is pivotal in setting the electron transport chain in motion. It delivers these electrons to the first protein complex of the ETC, Complex I.
-
FADH2: Similar to NADH, FADH2 is also generated during the Krebs cycle. However, it delivers its electrons to a later point in the ETC, Complex II, resulting in a slightly lower ATP yield.
These electrons, brimming with energy, are the driving force behind ATP production in the ETC.
The Electron Transport Chain: A Cascade of Redox Reactions
The ETC is embedded within the inner mitochondrial membrane in eukaryotes and the plasma membrane in prokaryotes. It's a series of protein complexes (I-IV) and mobile electron carriers (ubiquinone (Q) and cytochrome c) that facilitate the stepwise transfer of electrons. This transfer is a series of redox reactions – reduction (gain of electrons) and oxidation (loss of electrons).
Complex I: NADH Dehydrogenase
The journey begins at Complex I (NADH dehydrogenase). NADH delivers its high-energy electrons to Complex I, becoming oxidized (NAD+). These electrons are then passed along a series of electron carriers within Complex I, which utilizes the energy released to pump protons (H+) from the mitochondrial matrix across the inner mitochondrial membrane into the intermembrane space. This creates a proton gradient, a crucial element in ATP synthesis.
Complex II: Succinate Dehydrogenase
Complex II (succinate dehydrogenase), unlike Complex I, doesn't pump protons. It receives electrons from FADH2, a product of the Krebs cycle. These electrons are then passed to ubiquinone (Q). This bypasses the proton pumping step of Complex I, contributing to the lower ATP yield associated with FADH2.
Ubiquinone (Q): The Mobile Electron Carrier
Ubiquinone (Q), also known as coenzyme Q, is a lipid-soluble molecule that acts as a mobile electron carrier. It shuttles electrons from both Complex I and Complex II to Complex III.
Complex III: Cytochrome bc1 Complex
Complex III (cytochrome bc1 complex) receives electrons from ubiquinone. As electrons move through Complex III, more protons are pumped from the matrix to the intermembrane space, further bolstering the proton gradient.
Cytochrome c: Another Mobile Electron Carrier
Cytochrome c, a small, water-soluble protein, accepts electrons from Complex III and carries them to Complex IV.
Complex IV: Cytochrome c Oxidase
Complex IV (cytochrome c oxidase) is the final electron acceptor complex. It receives electrons from cytochrome c and ultimately transfers them to molecular oxygen (O2), the final electron acceptor, reducing it to water (H2O). This step also contributes to proton pumping, enhancing the proton gradient.
Chemiosmosis: The Proton Motive Force and ATP Synthase
The meticulously constructed proton gradient across the inner mitochondrial membrane is the key to ATP synthesis. This gradient represents stored energy, a proton motive force (PMF), that drives ATP production. The PMF consists of two components:
- Chemical gradient: The difference in proton concentration between the intermembrane space and the matrix.
- Electrical gradient: The difference in charge across the membrane, due to the movement of positively charged protons.
This PMF drives protons back across the inner mitochondrial membrane through a remarkable enzyme complex: ATP synthase.
ATP Synthase: The Molecular Turbine
ATP synthase is a remarkable molecular machine embedded in the inner mitochondrial membrane. It acts like a tiny turbine, harnessing the energy of the proton flow to synthesize ATP. Protons flowing through ATP synthase cause a rotation within the enzyme, driving conformational changes that catalyze the phosphorylation of adenosine diphosphate (ADP) to ATP. This is a crucial step in oxidative phosphorylation.
The ATP Yield: A Closer Look
The exact ATP yield from oxidative phosphorylation varies slightly depending on the shuttle system used to transport NADH from glycolysis to the mitochondria, but a general estimate is:
- Each NADH molecule generates approximately 2.5 ATP molecules.
- Each FADH2 molecule generates approximately 1.5 ATP molecules.
These figures take into account the efficiency of proton pumping and the stoichiometry of ATP synthase. The overall ATP yield from cellular respiration, including glycolysis and the Krebs cycle, is significantly higher than what can be generated through glycolysis alone.
Regulation of Oxidative Phosphorylation
The process of oxidative phosphorylation is tightly regulated to meet the cell's energy demands. Several factors influence its rate, including:
- ADP levels: High ADP levels signal a need for ATP synthesis, stimulating oxidative phosphorylation.
- Oxygen availability: Oxygen is the final electron acceptor, and its absence halts the ETC.
- Inhibitors: Certain molecules can inhibit specific complexes within the ETC, disrupting ATP production.
- Uncouplers: These molecules dissipate the proton gradient, reducing ATP synthesis but increasing heat production (e.g., in brown adipose tissue).
Clinical Significance: Mitochondrial Disorders
Dysfunctions in the ETC are implicated in a range of human diseases, collectively termed mitochondrial disorders. These disorders can manifest in diverse ways depending on the affected complex and the severity of the defect. They often present with symptoms related to energy deficiency in affected tissues, impacting the nervous system, muscles, and other organs.
Conclusion: The Powerhouse of the Cell
The electron transport chain and oxidative phosphorylation represent a marvel of cellular biochemistry. The intricate interplay of protein complexes, electron carriers, and proton gradients allows cells to extract substantial energy from nutrients, generating the ATP needed to fuel countless cellular processes. Understanding the details of this process is vital for appreciating the complexity and elegance of cellular respiration and the significance of its role in maintaining life. Further research continues to unveil the finer points of this process and its regulation, providing critical insights into human health and disease.
Latest Posts
Latest Posts
-
What Is The Shape Of Atom
May 11, 2025
-
Is Luster A Chemical Or Physical Property
May 11, 2025
-
How To Convert Kb To Ka
May 11, 2025
-
Draw The Fischer Projection For L Gulose
May 11, 2025
-
Is Mitochondria Found In Both Prokaryotic And Eukaryotic Cells
May 11, 2025
Related Post
Thank you for visiting our website which covers about During The Electron Transport Chain Atp Will Be Made From . We hope the information provided has been useful to you. Feel free to contact us if you have any questions or need further assistance. See you next time and don't miss to bookmark.