Electron Group Arrangement Vs Molecular Shape
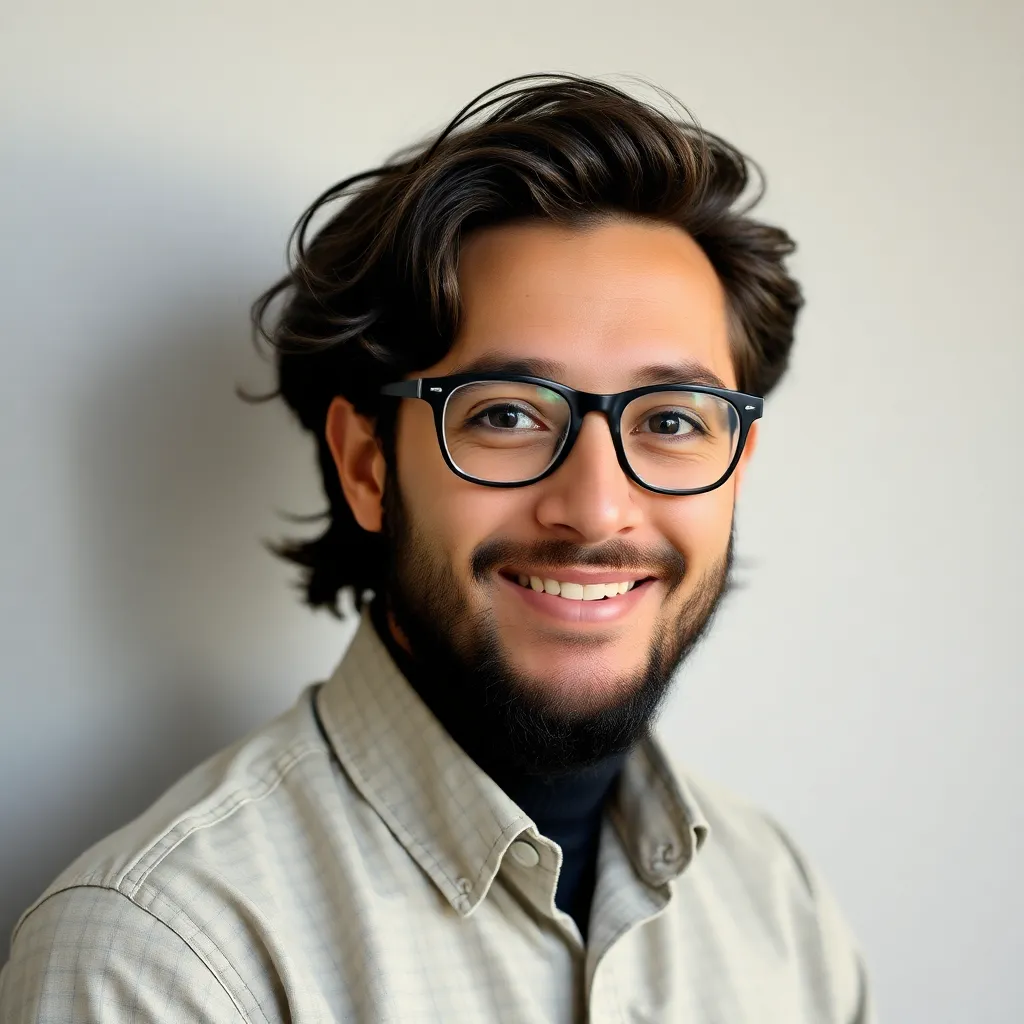
Muz Play
Mar 11, 2025 · 6 min read

Table of Contents
Electron Group Arrangement vs. Molecular Shape: A Comprehensive Guide
Understanding the three-dimensional structure of molecules is fundamental in chemistry. It dictates a molecule's properties, including its reactivity, polarity, and physical state. While seemingly complex, the arrangement of atoms within a molecule is governed by predictable principles rooted in the behavior of electrons. This article delves into the crucial distinction between electron group arrangement and molecular shape, explaining the underlying theories and providing numerous examples.
Understanding Electron Groups
Before diving into the differences, let's clarify what constitutes an "electron group." An electron group refers to any region of high electron density surrounding a central atom. This includes:
- Bonding pairs: These are pairs of electrons shared between the central atom and another atom, forming a covalent bond. Single, double, and triple bonds all count as one electron group.
- Lone pairs: These are pairs of electrons that are not involved in bonding and reside solely on the central atom. They significantly influence the molecule's shape.
The number of electron groups surrounding a central atom dictates the electron group arrangement, which is the ideal spatial distribution of these groups to minimize repulsion. This arrangement is predicted using the Valence Shell Electron Pair Repulsion (VSEPR) theory.
The VSEPR Theory: Predicting Electron Group Arrangement
The VSEPR theory is a cornerstone of molecular geometry prediction. It posits that electron groups around a central atom will arrange themselves to be as far apart as possible to minimize repulsive forces between them. This leads to several predictable geometries:
Key VSEPR Geometries Based on Electron Group Number:
-
2 Electron Groups (Linear): The electron groups are positioned 180° apart. Examples include BeCl₂ and CO₂.
-
3 Electron Groups (Trigonal Planar): The electron groups are arranged in a flat triangle with bond angles of approximately 120°. Examples include BF₃ and SO₃.
-
4 Electron Groups (Tetrahedral): The electron groups are positioned at the corners of a tetrahedron with bond angles of approximately 109.5°. Examples include CH₄ and SiCl₄.
-
5 Electron Groups (Trigonal Bipyramidal): This arrangement involves three equatorial electron groups (120° apart) and two axial electron groups (180° apart). Examples include PCl₅ and SF₄.
-
6 Electron Groups (Octahedral): The electron groups are arranged at the corners of an octahedron with bond angles of 90° and 180°. Examples include SF₆ and XeF₆.
Molecular Shape: The Influence of Lone Pairs
While electron group arrangement describes the positioning of all electron groups (bonding and lone pairs), molecular shape refers specifically to the arrangement of atoms in a molecule. Lone pairs, because they occupy more space than bonding pairs, exert a greater repulsive force. This causes deviations from the ideal electron group arrangements, leading to different molecular shapes.
Here's how lone pairs affect molecular shape for various electron group arrangements:
Molecular Shapes Derived from Different Electron Group Arrangements:
Let's consider the molecular shapes derived from each electron group arrangement, accounting for the presence of lone pairs:
-
2 Electron Groups:
- Linear (0 lone pairs): The molecular shape is linear, identical to the electron group arrangement (e.g., BeCl₂).
-
3 Electron Groups:
- Trigonal Planar (0 lone pairs): The molecular shape is trigonal planar, identical to the electron group arrangement (e.g., BF₃).
- Bent or Angular (1 or 2 lone pairs): The presence of one or two lone pairs causes the bond angle to decrease from 120° (e.g., SO₂, H₂O).
-
4 Electron Groups:
- Tetrahedral (0 lone pairs): The molecular shape is tetrahedral, identical to the electron group arrangement (e.g., CH₄).
- Trigonal Pyramidal (1 lone pair): The presence of one lone pair reduces the symmetry, resulting in a trigonal pyramidal shape (e.g., NH₃).
- Bent or Angular (2 lone pairs): Two lone pairs further reduce the symmetry, leading to a bent or angular shape (e.g., H₂O).
-
5 Electron Groups:
- Trigonal Bipyramidal (0 lone pairs): The molecular shape is trigonal bipyramidal, identical to the electron group arrangement (e.g., PCl₅).
- See-saw (1 lone pair): A lone pair in an equatorial position leads to a see-saw shape (e.g., SF₄).
- T-shaped (2 lone pairs): Two lone pairs in equatorial positions result in a T-shaped molecule (e.g., ClF₃).
- Linear (3 lone pairs): Three lone pairs in equatorial positions leave a linear arrangement of atoms (e.g., XeF₂).
-
6 Electron Groups:
- Octahedral (0 lone pairs): The molecular shape is octahedral, identical to the electron group arrangement (e.g., SF₆).
- Square Pyramidal (1 lone pair): One lone pair leads to a square pyramidal shape (e.g., BrF₅).
- Square Planar (2 lone pairs): Two lone pairs in opposite positions result in a square planar shape (e.g., XeF₄).
Distinguishing Electron Group Arrangement from Molecular Shape: Examples
Let's illustrate the difference with specific examples:
1. Methane (CH₄):
- Electron Group Arrangement: Tetrahedral (4 bonding pairs, 0 lone pairs)
- Molecular Shape: Tetrahedral (The arrangement of the H atoms mirrors the electron group arrangement)
2. Ammonia (NH₃):
- Electron Group Arrangement: Tetrahedral (3 bonding pairs, 1 lone pair)
- Molecular Shape: Trigonal Pyramidal (The lone pair affects the shape, pushing the hydrogen atoms closer together)
3. Water (H₂O):
- Electron Group Arrangement: Tetrahedral (2 bonding pairs, 2 lone pairs)
- Molecular Shape: Bent or Angular (The two lone pairs significantly distort the shape from tetrahedral)
Impact on Molecular Properties
The difference between electron group arrangement and molecular shape has significant implications for a molecule's properties:
-
Polarity: Molecular shape influences the molecule's overall dipole moment. Symmetrical molecules like CO₂ are nonpolar even though they have polar bonds, while asymmetrical molecules like H₂O are polar due to their bent shape.
-
Reactivity: The accessibility of atoms and lone pairs is determined by the molecular shape, influencing reaction pathways and rates.
-
Boiling Point and Melting Point: Molecular shape affects intermolecular forces (like hydrogen bonding and dipole-dipole interactions), consequently impacting boiling and melting points. Linear molecules generally have lower boiling points than similarly sized bent molecules due to weaker intermolecular forces.
-
Spectroscopic Properties: Molecular shape significantly affects the absorption and emission of electromagnetic radiation. This impacts techniques like infrared and Raman spectroscopy used for molecular structure determination.
Advanced Considerations and Limitations of VSEPR
While VSEPR theory provides a useful framework for predicting molecular geometries, it has limitations:
-
Complex Molecules: For large and complex molecules, predicting the precise shape can become challenging due to the increasing number of interactions and steric effects.
-
Transition Metal Complexes: VSEPR theory is less effective in predicting shapes of transition metal complexes, which often involve d-orbitals and ligand field effects.
-
Exceptions: Some molecules deviate from VSEPR predictions due to factors like the involvement of multiple bonds and the size of atoms.
Conclusion
Understanding the distinction between electron group arrangement and molecular shape is crucial for comprehending molecular behavior and properties. VSEPR theory offers a valuable tool for predicting these geometries, although its limitations should be acknowledged. By considering both the number and type of electron groups surrounding a central atom, we can accurately predict the molecular shape and understand its impact on a molecule’s physical and chemical characteristics. This knowledge forms the basis for advanced studies in various chemical disciplines. Remember that while VSEPR provides a strong predictive model, other methods like X-ray crystallography and computational chemistry are essential for confirming molecular structures and addressing the limitations of simpler models.
Latest Posts
Latest Posts
-
The Study Of Matter And The Changes It Undergoes
May 09, 2025
-
Colorimetric Determination Of An Equilibrium Constant In Aqueous Solution
May 09, 2025
-
How Does Electronegativity Affect Interactions Between Water Molecules
May 09, 2025
-
Using Ksp To Calculate The Solubility Of A Compound
May 09, 2025
-
Is Ethyl Alcohol Acidic Or Basic
May 09, 2025
Related Post
Thank you for visiting our website which covers about Electron Group Arrangement Vs Molecular Shape . We hope the information provided has been useful to you. Feel free to contact us if you have any questions or need further assistance. See you next time and don't miss to bookmark.