Electrons In The Outermost Principal Energy Level
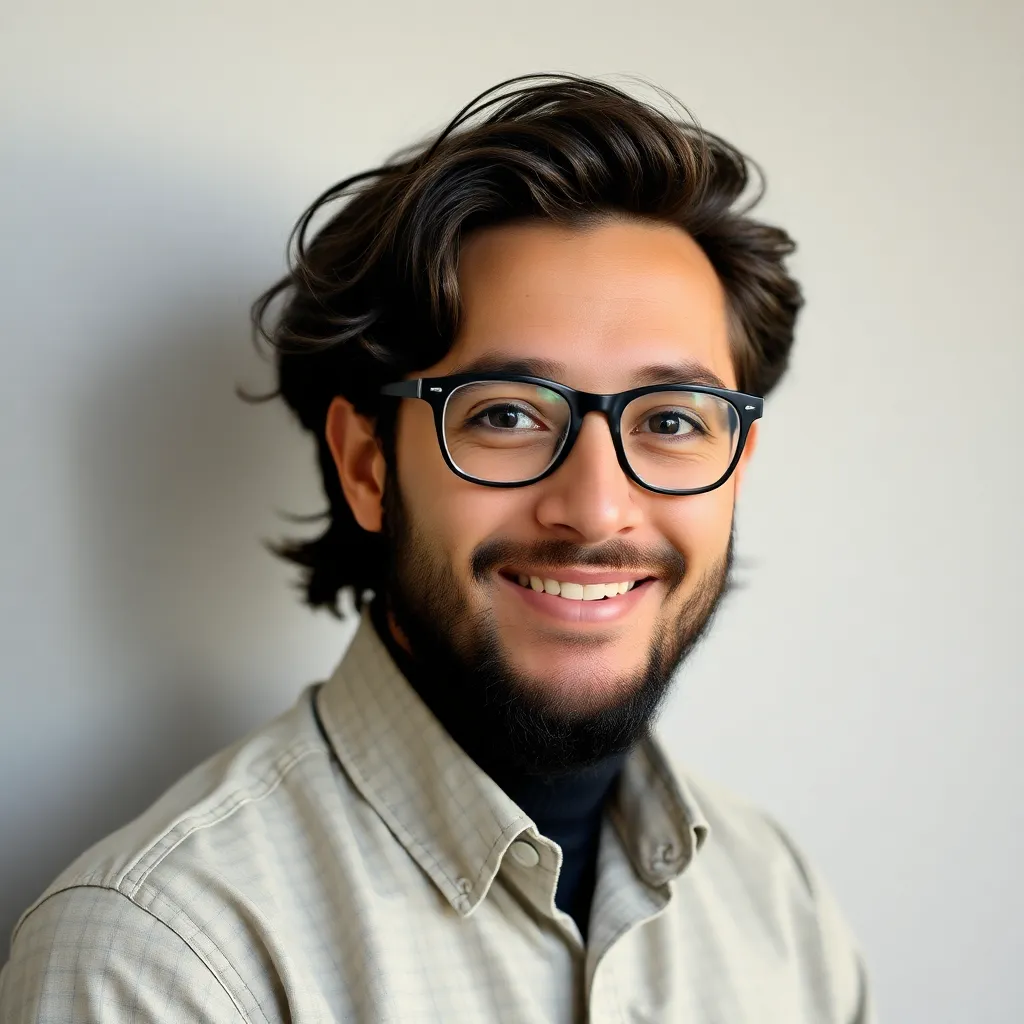
Muz Play
May 09, 2025 · 7 min read
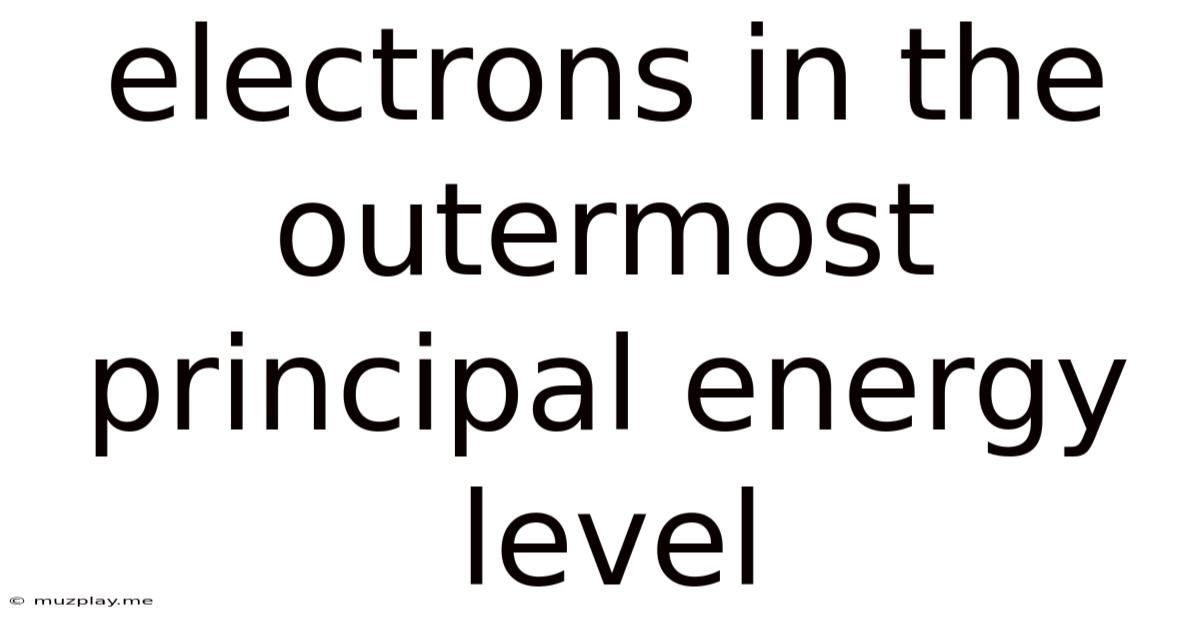
Table of Contents
Electrons in the Outermost Principal Energy Level: Valence Electrons and Their Chemical Prowess
Electrons, the fundamental negatively charged particles of an atom, dictate its chemical behavior. While all electrons contribute to an atom's overall properties, those residing in the outermost principal energy level, known as valence electrons, hold a particularly significant role. Understanding their behavior is crucial to comprehending chemical bonding, reactivity, and the periodic trends observed across the elements. This article delves deep into the world of valence electrons, exploring their characteristics, significance in chemical reactions, and their influence on various atomic and molecular properties.
Understanding Principal Energy Levels and Electron Shells
Before focusing on valence electrons, it's important to establish a foundational understanding of electron shells and principal energy levels. Electrons don't orbit the nucleus in random patterns; instead, they occupy specific energy levels, often visualized as concentric shells surrounding the nucleus. These energy levels are quantized, meaning electrons can only exist at certain discrete energy values. The principal quantum number (n) designates these energy levels, with n = 1 representing the lowest energy level (closest to the nucleus), n = 2 the next higher level, and so on. Each principal energy level can hold a maximum number of electrons, determined by the formula 2n². Thus, the first shell (n=1) can hold a maximum of 2 electrons, the second shell (n=2) a maximum of 8, the third shell (n=3) a maximum of 18, and so forth.
Electron Subshells and Orbitals
Within each principal energy level, electrons are further categorized into subshells, denoted by the letters s, p, d, and f. These subshells represent different shapes and orientations of atomic orbitals, which are regions of space where there's a high probability of finding an electron. The s subshell has one orbital, capable of holding two electrons; the p subshell has three orbitals, holding a total of six electrons; the d subshell has five orbitals, holding ten electrons; and the f subshell has seven orbitals, holding fourteen electrons. The filling of these subshells follows the Aufbau principle, which dictates that electrons fill the lowest energy levels first.
The Significance of Valence Electrons
The outermost principal energy level is where the valence electrons reside. These electrons are the most loosely bound to the nucleus and, therefore, the most readily involved in chemical interactions. They are the primary determinants of an element's chemical properties and reactivity. The number of valence electrons directly influences how an atom will bond with other atoms, forming molecules and compounds.
Determining the Number of Valence Electrons
For elements in groups 1 and 2 (alkali and alkaline earth metals), the number of valence electrons is simply the group number. For elements in groups 13-18 (main group elements), the number of valence electrons is equal to the group number minus 10. Transition metals (groups 3-12) have more complex valence electron configurations, and predicting their number requires a deeper understanding of their electron configurations. The noble gases (group 18) have full valence shells (typically eight electrons), making them exceptionally stable and unreactive. This stability is the driving force behind the formation of chemical bonds.
Valence Electrons and Chemical Bonding
The behavior of valence electrons is central to the formation of chemical bonds, the forces that hold atoms together in molecules and compounds. There are three primary types of chemical bonds:
1. Ionic Bonds
Ionic bonds form through the transfer of electrons from one atom to another. This transfer typically occurs between a metal (which tends to lose electrons readily) and a nonmetal (which tends to gain electrons readily). The resulting ions (charged atoms) are then held together by electrostatic attraction. For example, in sodium chloride (NaCl), sodium (Na) loses one valence electron to chlorine (Cl), forming Na⁺ and Cl⁻ ions, which are then attracted to each other, forming the ionic compound.
2. Covalent Bonds
Covalent bonds form through the sharing of electrons between two nonmetal atoms. Both atoms contribute valence electrons to form a shared electron pair, creating a bond between them. The shared electrons are attracted to the nuclei of both atoms, holding them together. For example, in methane (CH₄), carbon shares its four valence electrons with four hydrogen atoms, each contributing one electron to form four covalent bonds.
3. Metallic Bonds
Metallic bonds are found in metals, where valence electrons are delocalized, meaning they're not associated with any particular atom but rather move freely throughout the metal lattice. This sea of delocalized electrons accounts for the characteristic properties of metals, such as high electrical and thermal conductivity, malleability, and ductility.
Valence Electrons and Periodic Trends
The periodic table is organized based on the electronic configuration of elements, and the number of valence electrons plays a crucial role in establishing periodic trends.
1. Ionization Energy
Ionization energy is the energy required to remove an electron from an atom. Elements with fewer valence electrons generally have lower ionization energies because these electrons are less tightly bound to the nucleus.
2. Electronegativity
Electronegativity is a measure of an atom's ability to attract electrons in a chemical bond. Elements with a high number of valence electrons tend to have higher electronegativities because they strongly attract electrons to complete their outermost shell.
3. Atomic Radius
Atomic radius refers to the size of an atom. As you move across a period in the periodic table, the atomic radius generally decreases because the increasing nuclear charge pulls the valence electrons closer to the nucleus. Moving down a group, the atomic radius increases due to the addition of new electron shells.
Valence Electrons and Chemical Reactivity
The number and arrangement of valence electrons directly influence an element's chemical reactivity. Elements with partially filled valence shells are generally more reactive than those with completely filled or empty valence shells. For example, alkali metals (group 1) with one valence electron are highly reactive because they readily lose this electron to achieve a stable electron configuration. Halogens (group 17) with seven valence electrons are also highly reactive because they readily gain an electron to complete their outermost shell. Noble gases (group 18), on the other hand, are extremely unreactive due to their complete valence shells, showcasing their inherent stability.
Applications and Examples
The understanding of valence electrons has far-reaching applications across various scientific and technological fields. Here are a few examples:
-
Material Science: The properties of materials are heavily influenced by the valence electrons of their constituent atoms. Semiconductors, for instance, rely on controlled manipulation of valence electrons to achieve their unique electrical properties. Designing new materials with specific properties often involves tailoring the valence electron configurations of the constituent atoms.
-
Chemistry: Predicting the reactivity and bonding behavior of elements and molecules relies heavily on understanding valence electrons. This knowledge forms the basis for synthesizing new compounds and understanding chemical reactions.
-
Catalysis: Catalysts, substances that speed up chemical reactions, often function by interacting with the valence electrons of reactant molecules, thereby lowering the activation energy of the reaction. Understanding valence electron behavior is critical for designing and improving catalysts.
-
Spectroscopy: Spectroscopic techniques, which analyze the interaction of light with matter, provide valuable insights into valence electron configurations. Different elements and molecules absorb and emit light at specific wavelengths, providing information about their electronic structure and bonding.
Conclusion
Valence electrons, the electrons residing in the outermost principal energy level, are the key players in determining the chemical properties and reactivity of elements. Their behavior governs the formation of chemical bonds, influences periodic trends, and has significant implications across various scientific and technological applications. A thorough understanding of valence electrons is essential for comprehending the fundamental principles of chemistry and materials science, paving the way for advancements in diverse fields. Further exploration of advanced topics like molecular orbital theory and band theory provides an even more detailed understanding of the role of valence electrons in complex systems.
Latest Posts
Latest Posts
-
Chemical Sedimentary Rocks Form From Materials
May 11, 2025
-
How Are Matter And Elements Related
May 11, 2025
-
Which Formula Represents A Double Displacement Reaction
May 11, 2025
-
Label The Bones Of The Nasal Complex
May 11, 2025
-
Is Responsible For Directing The Synthesis Of Proteins
May 11, 2025
Related Post
Thank you for visiting our website which covers about Electrons In The Outermost Principal Energy Level . We hope the information provided has been useful to you. Feel free to contact us if you have any questions or need further assistance. See you next time and don't miss to bookmark.