Evaluate The Representation Of Ionic Bonding
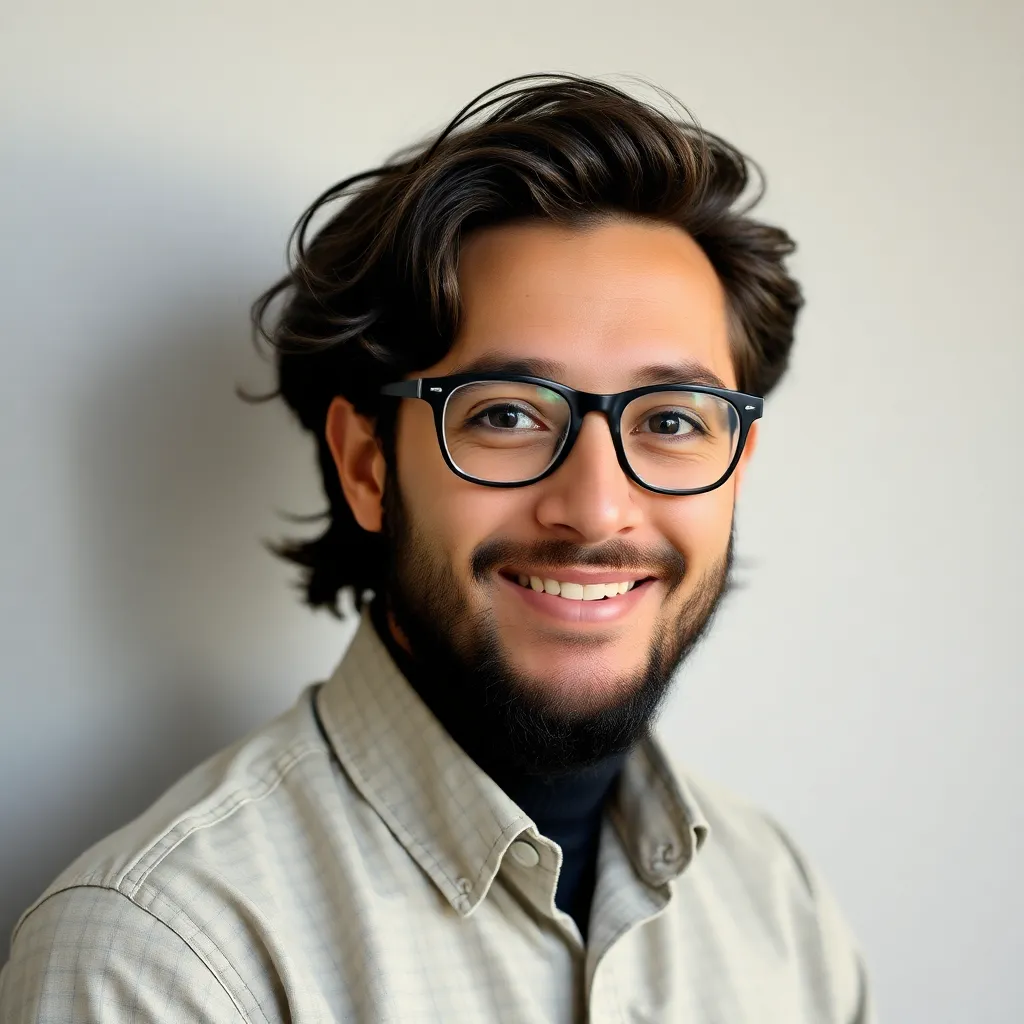
Muz Play
Apr 06, 2025 · 6 min read
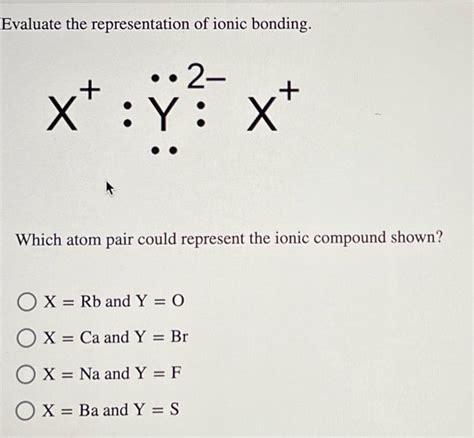
Table of Contents
Evaluating the Representation of Ionic Bonding: From Simple Models to Complex Realities
Ionic bonding, a fundamental concept in chemistry, describes the electrostatic attraction between oppositely charged ions. While the simple model of electron transfer and coulombic attraction provides a foundational understanding, a comprehensive evaluation reveals a far richer and more nuanced reality. This article delves into various representations of ionic bonding, examining their strengths and limitations, and highlighting the complexities that arise when moving beyond simplistic depictions.
The Simple Model: A Starting Point
The most basic representation of ionic bonding portrays a complete transfer of one or more electrons from a metal atom (electropositive) to a non-metal atom (electronegative). This transfer creates a positively charged cation and a negatively charged anion, held together by the strong electrostatic force of attraction. This model is often illustrated with Lewis dot structures, showing the transfer of valence electrons and the resulting charges.
Strengths:
- Simplicity and Ease of Understanding: The simple model provides a straightforward introduction to ionic bonding, easily grasped by beginners.
- Explains Basic Properties: It effectively explains some key properties of ionic compounds, such as high melting and boiling points (due to strong electrostatic forces), brittleness (due to repulsion of like charges upon shifting layers), and the ability to conduct electricity when molten or dissolved (due to the presence of mobile ions).
Limitations:
- Oversimplification of Electron Transfer: The complete transfer of electrons rarely occurs in reality. Electronegativity differences between atoms influence the degree of charge separation, with many ionic compounds exhibiting significant covalent character.
- Ignores Complex Interactions: The model fails to consider other interatomic forces, such as van der Waals forces, which also contribute to the overall stability of the ionic crystal lattice.
- Fails to Account for Polarization Effects: The model neglects the polarization of ions, where the electron cloud of an anion can be distorted by the presence of a nearby cation, leading to deviations from ideal ionic character.
Beyond the Simple Model: Incorporating Nuances
To gain a more accurate representation of ionic bonding, we must move beyond the simplistic picture of complete electron transfer. Several refinements and considerations help bridge the gap between the idealized model and the complexities of real ionic compounds:
1. Electronegativity and Covalent Character:
Electronegativity, a measure of an atom's ability to attract electrons in a chemical bond, plays a crucial role in determining the degree of ionic character. The greater the electronegativity difference between two atoms, the more ionic the bond. However, even in highly ionic compounds, some degree of electron sharing (covalent character) persists. This is particularly evident in compounds involving highly charged ions or ions with similar sizes. The concept of % ionic character, often calculated using Pauling's electronegativity scale, offers a quantitative assessment of this deviation from pure ionic bonding.
2. Lattice Energy and Born-Haber Cycle:
The stability of an ionic compound is determined by its lattice energy, the energy released when gaseous ions combine to form a solid crystal lattice. The Born-Haber cycle is a thermodynamic approach used to calculate the lattice energy, considering various energy changes, including ionization energies, electron affinities, and enthalpy of sublimation. The magnitude of the lattice energy reflects the strength of the ionic bond, offering a more quantitative perspective than simply invoking Coulomb's law. Higher lattice energy generally implies stronger bonding and greater stability.
3. Crystal Structure and Coordination Number:
The arrangement of ions in a crystal lattice, described by its crystal structure (e.g., cubic close-packed, face-centered cubic), significantly influences the stability and properties of the ionic compound. The coordination number, representing the number of ions surrounding a central ion, is crucial in determining the packing efficiency and overall lattice energy. Different crystal structures result in variations in the distances between ions and thus the strength of electrostatic interactions.
4. Polarization and Distortions of Ion Shapes:
The electron cloud of an ion isn't static; it can be distorted or polarized by the electric field of a neighboring ion. This polarization effect is more pronounced when the cation is small and highly charged (high charge density) and the anion is large and easily polarizable. Polarization reduces the ionic character of the bond and can even lead to the formation of covalent bonds. Fajan's rules provide guidelines for predicting the extent of polarization and its influence on the properties of ionic compounds.
Advanced Representations: Beyond Simple Models
Moving beyond the limitations of simple models requires incorporating advanced techniques and concepts:
1. Molecular Orbital Theory (MOT):
While primarily used for covalent bonding, MOT can be applied to ionic compounds to provide a more accurate description of electron distribution. MOT considers the interactions of atomic orbitals to form molecular orbitals, encompassing both bonding and antibonding orbitals. In ionic compounds, this approach can reveal the degree of electron delocalization and the extent of covalent character.
2. Density Functional Theory (DFT):
DFT is a powerful computational technique used to study the electronic structure of molecules and solids. It can provide accurate predictions of lattice energies, bond lengths, and other properties of ionic compounds. DFT calculations account for electron correlation and exchange effects, going beyond the limitations of simpler models. They provide a detailed, quantitative understanding of the electron distribution and bonding interactions in ionic crystals.
3. Experimental Techniques:
Experimental techniques like X-ray crystallography, neutron diffraction, and spectroscopy offer valuable insights into the structure and bonding in ionic compounds. X-ray crystallography determines the precise arrangement of ions in the crystal lattice, providing information on bond lengths and coordination numbers. Spectroscopic techniques (e.g., infrared, Raman, NMR) provide information on vibrational modes and electronic transitions, which can be used to infer the nature of bonding interactions.
Case Studies: Examining Specific Examples
Analyzing specific examples helps to illustrate the complexities of ionic bonding representation. Consider:
-
Sodium Chloride (NaCl): While often presented as a purely ionic compound, NaCl exhibits a small degree of covalent character due to polarization effects. DFT calculations show a slight deviation from complete charge transfer, reflecting the complexities beyond the simple model.
-
Lithium Fluoride (LiF): LiF is a highly ionic compound due to the large electronegativity difference between Li and F. However, the small size of the Li⁺ cation leads to significant polarization of the F⁻ anion, again illustrating the importance of considering polarization effects.
-
Transition Metal Compounds: Transition metal compounds frequently display significant covalent character due to the participation of d-orbitals in bonding. Their bonding is often better described using a combination of ionic and covalent models, highlighting the limitations of a purely ionic representation.
Conclusion: The Importance of a Nuanced Approach
The representation of ionic bonding evolves from a simple model of complete electron transfer to a more nuanced understanding incorporating electronegativity differences, lattice energy, crystal structure, polarization effects, and advanced computational techniques. While the simple model offers a foundational understanding, it is crucial to acknowledge its limitations and appreciate the complexities inherent in the behavior of real ionic compounds. A comprehensive understanding requires a multi-faceted approach, integrating theoretical models with experimental observations to provide a realistic and accurate representation of these fundamental chemical interactions. The use of advanced computational methods like DFT offers increasingly sophisticated tools to study these intricate interactions, further enhancing our understanding of the fascinating world of ionic bonding.
Latest Posts
Latest Posts
-
How Do New Heritable Traits Arise In A Population
Apr 06, 2025
-
Vsper Theory Is Used To Predict The
Apr 06, 2025
-
Is Eosin Methylene Blue Agar Selective Or Differential
Apr 06, 2025
-
Write The Standard Form Of The Equation Of Each Line
Apr 06, 2025
-
The Law Of Segregation Explains That
Apr 06, 2025
Related Post
Thank you for visiting our website which covers about Evaluate The Representation Of Ionic Bonding . We hope the information provided has been useful to you. Feel free to contact us if you have any questions or need further assistance. See you next time and don't miss to bookmark.