Every Chemical Reaction Involves A Change In
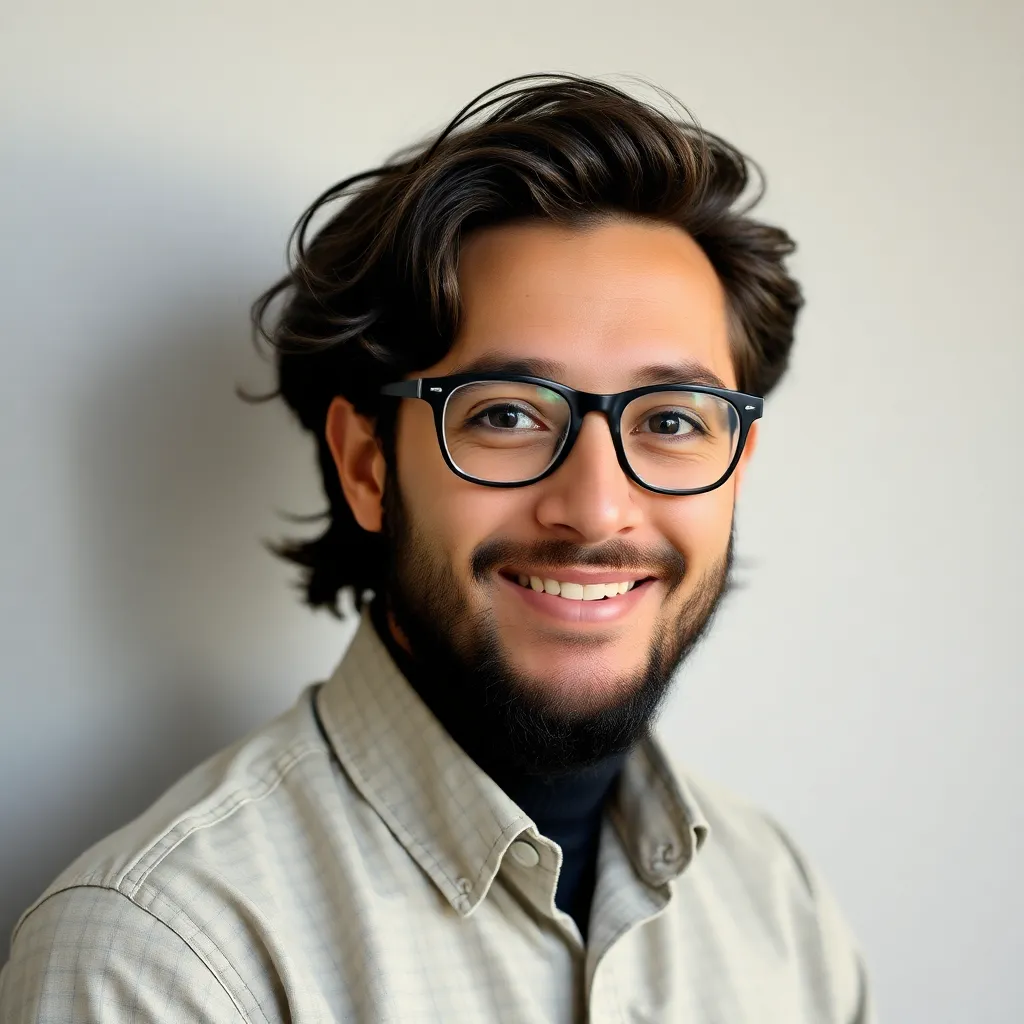
Muz Play
Apr 18, 2025 · 7 min read

Table of Contents
Every Chemical Reaction Involves a Change in: A Deep Dive into Energy, Matter, and Entropy
Chemical reactions are the fundamental building blocks of the universe, shaping everything from the formation of stars to the processes within our own bodies. Understanding these reactions requires grasping a core principle: every chemical reaction involves a change in energy, matter, and entropy. This article delves deep into each of these changes, exploring the intricate relationships between them and providing examples to illustrate their significance.
The Inevitable Change in Energy
One of the most fundamental aspects of any chemical reaction is the change in energy. This energy change can manifest in various forms, primarily as:
1. Heat (Enthalpy Change, ΔH):
Many chemical reactions release or absorb heat. Reactions that release heat to their surroundings are called exothermic reactions (ΔH < 0), while those that absorb heat are endothermic reactions (ΔH > 0). The amount of heat exchanged is crucial for understanding the reaction's feasibility and spontaneity. A classic example of an exothermic reaction is the combustion of methane (natural gas), releasing a significant amount of heat in the process. Conversely, the melting of ice is an endothermic process, requiring heat input to overcome the intermolecular forces holding the water molecules together.
2. Light (Photochemical Reactions):
Some chemical reactions involve the absorption or emission of light. These are known as photochemical reactions. Photosynthesis is a prime example, where plants absorb light energy to convert carbon dioxide and water into glucose and oxygen. The energy from sunlight drives this endothermic process, initiating a complex series of chemical transformations. Conversely, certain chemical reactions, like chemiluminescence (e.g., glow sticks), release energy as light.
3. Electrical Energy (Electrochemical Reactions):
Electrochemical reactions involve the transfer of electrons, leading to a change in electrical energy. Batteries are a practical application of this principle, where chemical reactions produce a flow of electrons, generating electrical energy. Conversely, electrolysis, used in processes like metal refining, utilizes electrical energy to drive non-spontaneous chemical reactions.
4. Work (Mechanical Energy):
Chemical reactions can also perform mechanical work. The expansion of gases during an explosion, for instance, results in mechanical work being done. Similarly, the contraction of muscles relies on chemical reactions that generate mechanical force, enabling movement.
Understanding the energy change in a reaction is crucial for predicting its spontaneity and determining the equilibrium position. Thermodynamics provides the framework for quantifying and analyzing these energy changes, using concepts like enthalpy, entropy, and Gibbs free energy.
The Transformation of Matter: Conservation of Mass
Another fundamental aspect of every chemical reaction is the change in matter. However, this change isn't about creation or destruction; rather, it's about rearrangement. The law of conservation of mass dictates that matter cannot be created or destroyed in a chemical reaction; it only changes form.
This means that the total mass of the reactants must equal the total mass of the products. Consider the combustion of methane:
CH₄ (methane) + 2O₂ (oxygen) → CO₂ (carbon dioxide) + 2H₂O (water)
The total mass of methane and oxygen before the reaction equals the total mass of carbon dioxide and water after the reaction. The atoms are simply rearranged to form new molecules.
While the law of conservation of mass holds true for most chemical reactions under ordinary conditions, it needs slight modification when considering nuclear reactions, where mass can be converted into energy (and vice-versa) according to Einstein's famous equation, E=mc².
The Arrow of Time: The Inevitable Increase in Entropy
The third crucial aspect of every chemical reaction is the change in entropy (ΔS). Entropy is a measure of disorder or randomness within a system. The second law of thermodynamics states that the total entropy of an isolated system can only increase over time or remain constant in ideal cases where the system is in a steady state or undergoing a reversible process. In simpler terms, systems tend towards disorder.
Most chemical reactions lead to an increase in entropy (ΔS > 0). Consider dissolving salt in water. The highly ordered crystalline structure of salt breaks down into individual ions dispersed randomly throughout the water. This represents an increase in disorder, thus an increase in entropy.
However, some reactions might show a decrease in entropy locally (ΔS < 0). For instance, the formation of a crystal from a solution involves a decrease in disorder. However, even in these cases, the overall entropy of the universe (including the surroundings) still increases. The energy released during crystallization often increases the disorder of the surroundings (e.g., by heating them), outweighing the local decrease in entropy within the crystal itself.
The change in entropy is crucial for understanding the spontaneity of a reaction. A reaction will be spontaneous if it leads to an increase in the overall entropy of the universe, even if it involves a local decrease in entropy.
The Interplay of Energy, Matter, and Entropy: Gibbs Free Energy
The interplay of energy (enthalpy, ΔH), matter (mass conservation), and entropy (ΔS) is best understood through the concept of Gibbs free energy (ΔG). Gibbs free energy is a thermodynamic potential that can be used to predict the spontaneity of a reaction at constant temperature and pressure. It's defined as:
ΔG = ΔH - TΔS
where:
-
ΔG is the change in Gibbs free energy
-
ΔH is the change in enthalpy
-
T is the temperature in Kelvin
-
ΔS is the change in entropy
-
If ΔG < 0: The reaction is spontaneous under the given conditions.
-
If ΔG > 0: The reaction is non-spontaneous under the given conditions. It requires energy input to proceed.
-
If ΔG = 0: The reaction is at equilibrium. The rates of the forward and reverse reactions are equal.
The Gibbs free energy equation elegantly captures the interplay between enthalpy and entropy in determining reaction spontaneity. A reaction with a negative ΔH (exothermic) and a positive ΔS (increase in disorder) will always be spontaneous (ΔG will always be negative). However, reactions with positive ΔH (endothermic) can still be spontaneous if the increase in entropy (TΔS) is large enough to outweigh the positive ΔH.
Real-World Applications and Examples
The principles of energy, matter, and entropy changes in chemical reactions have far-reaching applications across various fields:
-
Industrial Chemistry: Optimizing industrial processes requires understanding the energy changes involved to minimize energy consumption and maximize yield. For example, the Haber-Bosch process for ammonia synthesis involves significant energy input to overcome the high activation energy of the reaction.
-
Biochemistry: Metabolic processes within living organisms involve countless chemical reactions, each characterized by changes in energy, matter, and entropy. Understanding these changes is fundamental to comprehending how life functions at a molecular level. ATP hydrolysis, for example, releases energy that fuels many cellular processes.
-
Environmental Science: Chemical reactions play a critical role in environmental processes, such as pollution formation and remediation. Understanding these reactions is crucial for developing strategies for environmental protection. For example, the combustion of fossil fuels releases greenhouse gases, contributing to climate change.
-
Materials Science: The synthesis of new materials often involves carefully controlling chemical reactions to obtain desired properties. Understanding the energy changes and entropy involved allows for the design of materials with specific functionalities. For instance, the synthesis of polymers involves reactions that lead to the formation of long chains, affecting the material's mechanical properties.
-
Medicine: Drug development and action are deeply rooted in chemical reactions. Understanding how drugs interact with biological systems at a molecular level is essential for designing effective therapies. Enzyme-catalyzed reactions are crucial in many biological processes and drug actions.
Conclusion
In conclusion, every chemical reaction involves a change in energy, matter, and entropy. These changes are interconnected and governed by the laws of thermodynamics. Understanding these fundamental principles is crucial for comprehending the behavior of chemical systems and for advancing our knowledge in various scientific and technological fields. By analyzing enthalpy, entropy, and Gibbs free energy changes, we can predict the spontaneity of reactions, optimize industrial processes, design new materials, and develop effective therapies, highlighting the universal importance of these principles in our world.
Latest Posts
Latest Posts
-
According To Bronsted Lowry Theory An Acid Is
Apr 19, 2025
-
Does The Entropy Of The Surroundings Increase For Spontaneous Processes
Apr 19, 2025
-
Construct An Mo Diagram For The He 2 Ion
Apr 19, 2025
-
Can The Zero Vector Be An Eigenvector
Apr 19, 2025
-
Concept Map Blood Groups And Transfusions
Apr 19, 2025
Related Post
Thank you for visiting our website which covers about Every Chemical Reaction Involves A Change In . We hope the information provided has been useful to you. Feel free to contact us if you have any questions or need further assistance. See you next time and don't miss to bookmark.