Example Of Voltage Gated Ion Channel
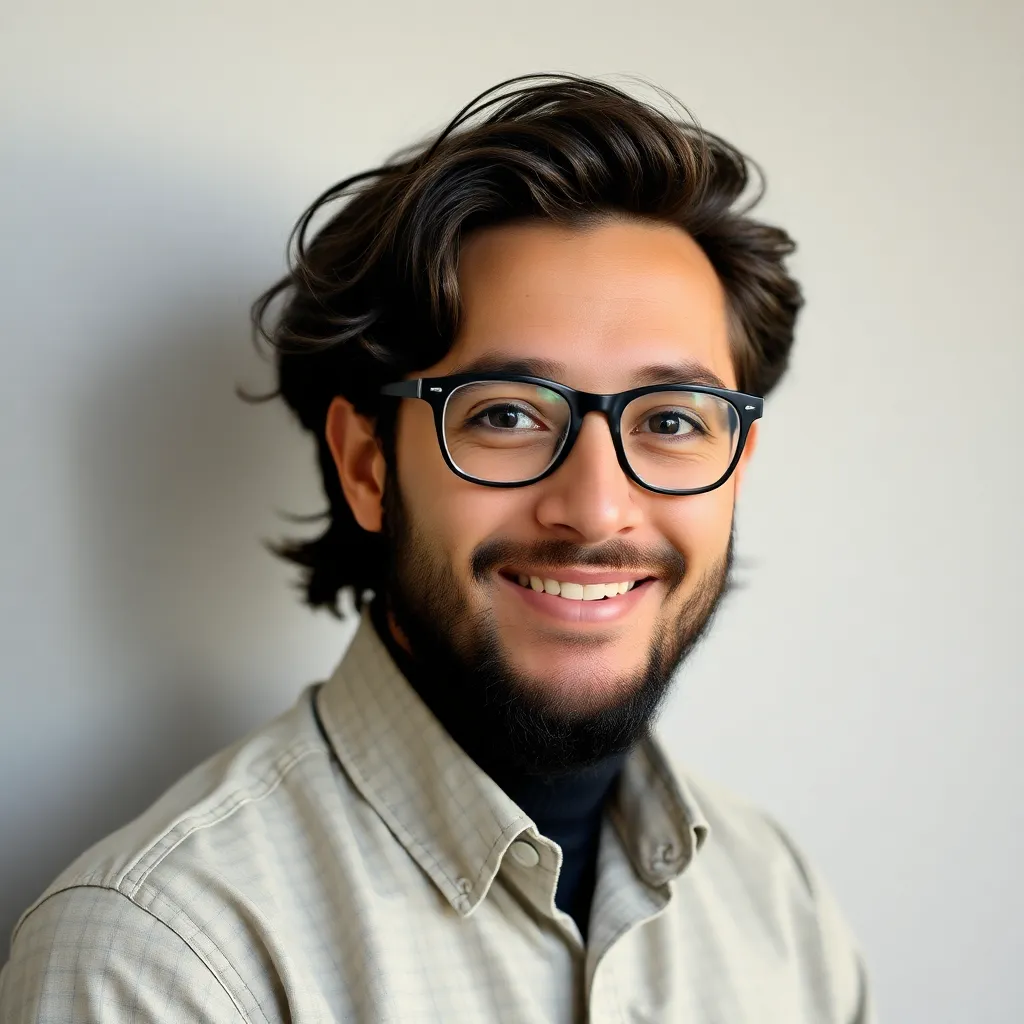
Muz Play
Apr 22, 2025 · 6 min read

Table of Contents
Voltage-Gated Ion Channels: Examples and Mechanisms
Voltage-gated ion channels are transmembrane proteins that play a crucial role in the electrical excitability of cells. They act as selective pores, allowing specific ions like sodium (Na+), potassium (K+), calcium (Ca2+), and chloride (Cl-) to flow across the cell membrane in response to changes in the membrane potential. This intricate mechanism underlies a vast array of physiological processes, including nerve impulse transmission, muscle contraction, and hormone secretion. This article delves into the fascinating world of voltage-gated ion channels, examining various examples and their underlying mechanisms in detail.
The Structure and Function of Voltage-Gated Ion Channels
Before exploring specific examples, it's crucial to understand the general structure and function of these channels. Voltage-gated ion channels are typically composed of four homologous domains, each containing six transmembrane α-helices (S1-S6). The S4 segment is particularly important; it acts as the voltage sensor, containing positively charged amino acid residues that respond to changes in the membrane potential. Depolarization (a decrease in membrane potential negativity) causes the S4 segment to move, inducing conformational changes that open the channel pore, allowing ion flow. Repolarization (return to resting membrane potential) reverses this process, closing the channel.
Examples of Voltage-Gated Ion Channels: A Deep Dive
The diversity of voltage-gated ion channels is vast, with different subtypes exhibiting unique properties in terms of ion selectivity, gating kinetics, and pharmacological sensitivity. Below, we explore several prominent examples:
1. Voltage-Gated Sodium Channels (Na<sub>v</sub> Channels): The Key Players in Action Potentials
Na<sub>v</sub> channels are perhaps the most well-known voltage-gated ion channels. Their rapid activation and inactivation are crucial for the initiation and propagation of action potentials in neurons and muscle cells. A significant depolarization opens the channels, leading to a massive influx of Na+ ions, which rapidly depolarizes the membrane. The channel then inactivates, preventing further Na+ influx and allowing repolarization to occur. Different subtypes of Na<sub>v</sub> channels exist, exhibiting variations in their kinetic properties and expression patterns, contributing to the diversity of neuronal and muscle functions. For instance, Na<sub>v</sub>1.5 is predominantly found in the heart, playing a critical role in cardiac action potential generation.
2. Voltage-Gated Potassium Channels (K<sub>v</sub> Channels): The Guardians of Resting Potential
K<sub>v</sub> channels are essential for maintaining the resting membrane potential and repolarizing the membrane after an action potential. They exhibit a diverse range of gating kinetics and are crucial for regulating the duration and frequency of action potentials. Several K<sub>v</sub> channel subtypes exist, each with distinct biophysical properties. Some K<sub>v</sub> channels activate rapidly after depolarization, contributing to the repolarization phase of the action potential. Others activate more slowly, contributing to the afterhyperpolarization, a period of hyperpolarization following the action potential. The diversity of these channels allows fine-tuning of electrical signaling in various cell types.
Specific examples of K<sub>v</sub> channels:
- K<sub>v</sub>1 channels: Known for their rapid activation and inactivation kinetics, playing a significant role in repolarization.
- K<sub>v</sub>4 channels: These channels contribute to the transient outward potassium current, influencing the shape and duration of action potentials.
- K<sub>v</sub>7 channels: Often referred to as M-channels, these channels are known for their slow activation and deactivation kinetics, influencing neuronal excitability.
3. Voltage-Gated Calcium Channels (Ca<sub>v</sub> Channels): Essential for Excitation-Contraction Coupling and Neurotransmitter Release
Ca<sub>v</sub> channels are crucial for a wide range of cellular processes, including muscle contraction, neurotransmitter release, and gene expression. Unlike Na<sub>v</sub> channels which have a fast inactivation mechanism, Ca<sub>v</sub> channels generally exhibit slower activation and inactivation kinetics, contributing to prolonged calcium influx. The entry of Ca2+ ions through these channels triggers various downstream effects, depending on the cell type. In muscle cells, Ca2+ influx initiates muscle contraction, while in neurons, it triggers neurotransmitter release at synapses.
Subtypes of Ca<sub>v</sub> channels:
- L-type Ca<sub>v</sub> channels: Known for their long-lasting activation, these channels are involved in excitation-contraction coupling in cardiac muscle and smooth muscle.
- T-type Ca<sub>v</sub> channels: These channels activate at more negative membrane potentials and exhibit rapid inactivation. They play a role in neuronal rhythmical firing and pacemaker activity.
- N-type Ca<sub>v</sub> channels: Primarily found in neurons, these channels mediate neurotransmitter release and are targets for various analgesic drugs.
- P/Q-type Ca<sub>v</sub> channels: These channels are also involved in neurotransmitter release at synapses.
4. Voltage-Gated Chloride Channels (Cl<sub>v</sub> Channels): Modulating Excitability
Cl<sub>v</sub> channels are less extensively studied compared to Na<sub>v</sub>, K<sub>v</sub>, and Ca<sub>v</sub> channels, but they play significant roles in regulating neuronal excitability and controlling cell volume. Opening of Cl<sub>v</sub> channels allows Cl- ions to flow across the membrane, influencing the membrane potential. Depending on the intracellular and extracellular chloride concentrations, this can lead to either hyperpolarization or depolarization. The role of Cl<sub>v</sub> channels in various physiological processes is still an active area of research.
Modulation of Voltage-Gated Ion Channels
The function of voltage-gated ion channels is tightly regulated by various factors, including:
- Phosphorylation: Protein kinases can phosphorylate ion channels, altering their gating kinetics and sensitivity to voltage changes.
- G-protein coupled receptors: Activation of G-protein coupled receptors can influence ion channel activity via direct or indirect mechanisms.
- Drugs and toxins: Many drugs and toxins target voltage-gated ion channels, altering their function and producing pharmacological effects. For example, local anesthetics block Na<sub>v</sub> channels, while some antiarrhythmic drugs target K<sub>v</sub> channels.
Clinical Significance of Voltage-Gated Ion Channels
Dysfunction of voltage-gated ion channels is implicated in a wide range of diseases, including:
- Cardiac arrhythmias: Mutations in genes encoding cardiac ion channels can cause life-threatening arrhythmias.
- Epilepsy: Abnormal neuronal excitability, often linked to ion channel dysfunction, is a hallmark of epilepsy.
- Muscle disorders: Myotonia and other muscle disorders can arise from defects in muscle ion channels.
- Neurological disorders: Certain neurological diseases are associated with altered ion channel function.
Future Directions
Research on voltage-gated ion channels continues to be a vibrant field. Ongoing studies focus on uncovering the precise mechanisms of channel regulation, identifying novel channel subtypes, and exploring their therapeutic potential in treating various diseases. Advanced techniques such as cryo-electron microscopy are providing unprecedented structural insights into these crucial proteins, paving the way for the development of more effective and targeted therapies. The exploration of the role of voltage-gated ion channels in diseases remains a critical area of focus, promising breakthroughs in diagnosis and treatment.
Conclusion
Voltage-gated ion channels are fundamental components of cellular excitability, playing essential roles in diverse physiological processes. Their intricate mechanisms, diverse subtypes, and susceptibility to modulation make them fascinating targets for research and drug development. Further exploration of these channels will undoubtedly lead to a deeper understanding of cellular function and improved treatments for a wide range of diseases. The examples provided here only represent a fraction of the numerous voltage-gated ion channels found in nature, showcasing the remarkable complexity and importance of these remarkable proteins.
Latest Posts
Latest Posts
-
What Refers To The Division Of The Nucleus
Apr 22, 2025
-
Electrons Travel In Paths Called Energy Levels
Apr 22, 2025
-
Does Sodium Lose Or Gain Electrons
Apr 22, 2025
-
What Is The Difference Between Base Units And Derived Units
Apr 22, 2025
-
Osmotic Pressure Is Measured In Units Of
Apr 22, 2025
Related Post
Thank you for visiting our website which covers about Example Of Voltage Gated Ion Channel . We hope the information provided has been useful to you. Feel free to contact us if you have any questions or need further assistance. See you next time and don't miss to bookmark.