Explain How An Enzyme Works Using The Terms From 1-5
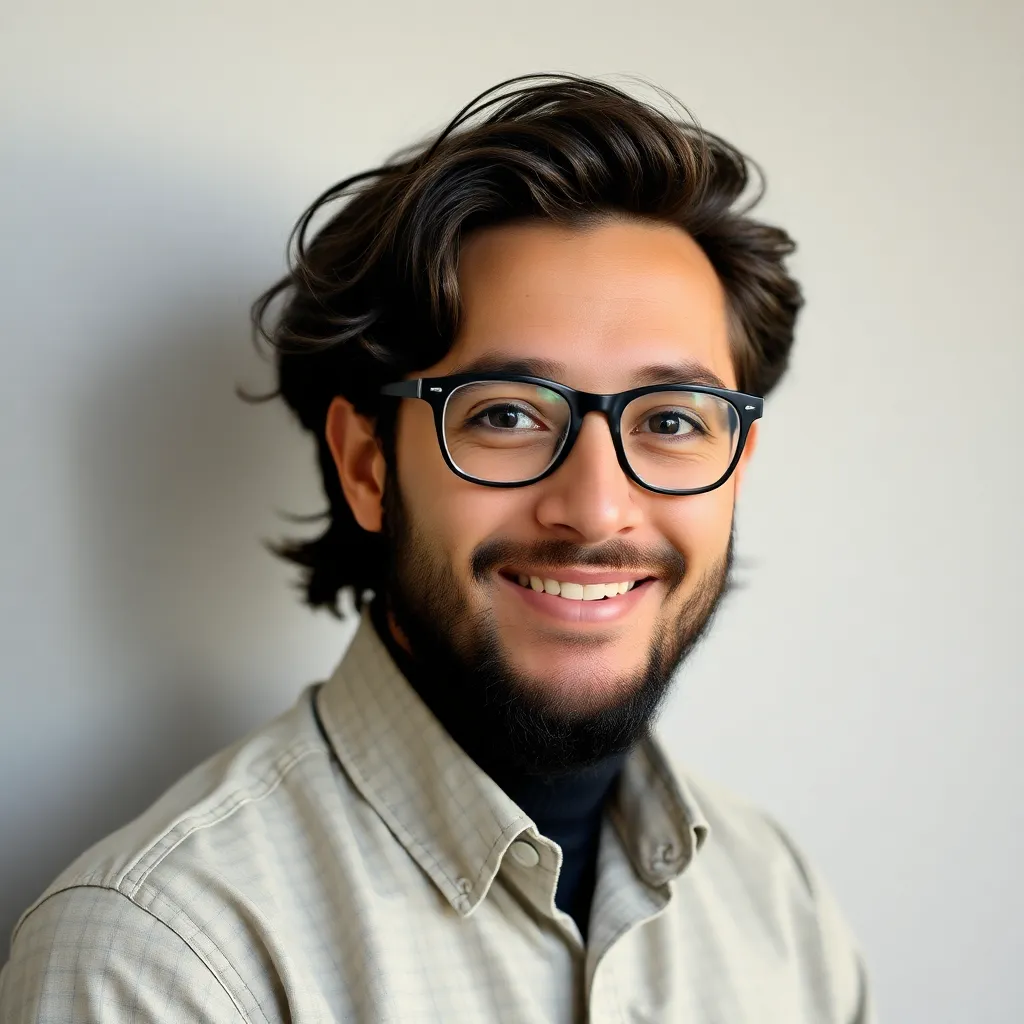
Muz Play
May 09, 2025 · 6 min read
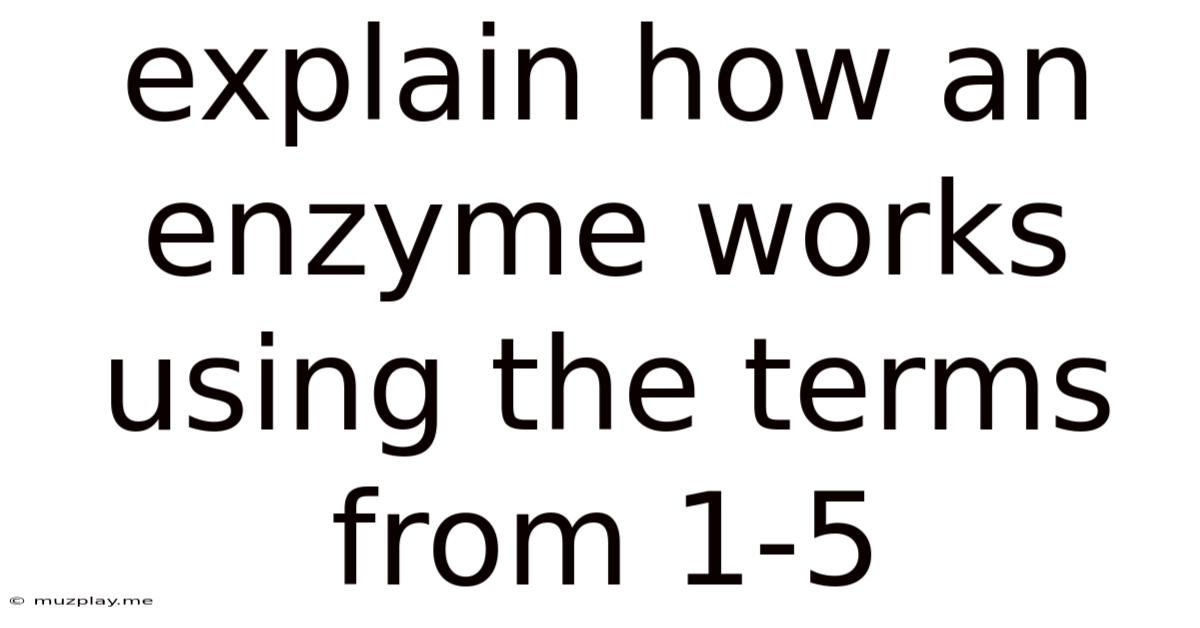
Table of Contents
How Enzymes Work: A Deep Dive into Catalytic Mechanisms
Enzymes are biological catalysts, crucial for virtually every biochemical reaction within living organisms. Understanding how they function is key to comprehending the intricacies of life itself. This article will explore the mechanism of enzyme action, employing a numbered approach to break down the process into manageable steps. We will also delve into the concepts of enzyme specificity, activation energy, and the factors influencing enzyme activity.
1. Substrate Binding and Enzyme Specificity: The Lock and Key Model (and its limitations)
The first step in enzyme catalysis involves the binding of the substrate – the molecule upon which the enzyme acts – to the active site. The active site is a specific three-dimensional region within the enzyme's structure, often a cleft or pocket, uniquely shaped to accommodate the substrate. This interaction is often described using the lock and key model, where the substrate (the key) precisely fits into the active site (the lock). This model, while conceptually simple, is an oversimplification.
Enzyme specificity is a crucial aspect of this initial binding. Enzymes are highly selective, generally catalyzing only a single type of reaction or a small group of closely related reactions. This selectivity arises from the precise three-dimensional arrangement of amino acid residues within the active site. Only substrates with the appropriate shape, charge distribution, and chemical functionalities can effectively bind and interact.
However, the lock and key model has limitations. It doesn't fully explain the induced fit mechanism, a refinement of the model.
The Induced Fit Model: A More Realistic Picture
The induced fit model proposes that the enzyme's active site is not a rigid, pre-formed structure but rather undergoes conformational changes upon substrate binding. The substrate's approach induces a change in the active site's shape, creating a more complementary and tighter binding interaction. This conformational change optimally positions catalytic groups within the active site, maximizing the efficiency of the catalytic process. This dynamic interaction is more accurate than the static lock and key model and explains the enzyme's ability to accommodate a range of similar substrates.
2. Transition State Stabilization: Lowering the Activation Energy
Once the substrate is bound to the active site, the enzyme facilitates the conversion of the substrate into products. This conversion doesn't occur spontaneously at a significant rate; it requires overcoming an energy barrier called the activation energy (Ea). Enzymes significantly lower this activation energy, thus accelerating the reaction rate. They achieve this primarily by stabilizing the transition state.
The transition state is a high-energy, unstable intermediate formed during the conversion of substrate to product. It's the point of highest energy along the reaction pathway. Enzymes achieve transition state stabilization through several mechanisms:
- Proximity and Orientation: Enzymes bring reactants together in close proximity and in the correct orientation, increasing the probability of successful collisions and facilitating the formation of the transition state.
- Acid-Base Catalysis: Amino acid residues within the active site can act as acids or bases, donating or accepting protons to facilitate bond breaking and formation in the transition state.
- Covalent Catalysis: The enzyme's active site can form temporary covalent bonds with the substrate, creating a more stable intermediate that lowers the activation energy.
- Metal Ion Catalysis: Many enzymes require metal ions as cofactors. These ions can participate in redox reactions, stabilize negative charges, or bridge between the enzyme and substrate, all contributing to transition state stabilization.
The lowering of activation energy is the fundamental reason why enzymes are so effective at speeding up reactions. They dramatically increase the rate of reaction without being consumed in the process.
3. Catalysis and Product Formation: The Reaction Mechanism
The exact mechanism of catalysis varies greatly depending on the specific enzyme and the reaction it catalyzes. However, several common themes emerge. After transition state stabilization, the enzyme facilitates the formation of the products. This may involve bond breaking, bond formation, or both.
Once the products are formed, they have a lower affinity for the active site than the substrate or transition state. This difference in binding affinity is crucial for product release, the final step in the catalytic cycle. Product release regenerates the free enzyme, ready to bind another substrate molecule and repeat the cycle.
4. Enzyme Kinetics: Measuring Enzyme Activity
The study of enzyme kinetics provides valuable insights into the catalytic mechanism and efficiency of enzymes. Key parameters used to describe enzyme activity include:
- Maximum Velocity (Vmax): The maximum rate of the reaction achieved at saturating substrate concentrations. At Vmax, all enzyme molecules are actively engaged in catalysis.
- Michaelis Constant (Km): The substrate concentration at which the reaction velocity is half of Vmax. Km provides an indication of the enzyme's affinity for its substrate; a lower Km indicates higher affinity.
- Turnover Number (kcat): The number of substrate molecules converted to product per enzyme molecule per unit time. It represents the enzyme's catalytic efficiency.
These parameters are often determined experimentally using methods such as the Michaelis-Menten equation and Lineweaver-Burk plots. Understanding these kinetic parameters provides crucial information regarding the enzyme's performance and can be used to model enzyme activity under various conditions.
5. Factors Affecting Enzyme Activity: Regulation and Control
Enzyme activity is not static; it's highly regulated to maintain cellular homeostasis and respond to changing environmental conditions. Several factors influence enzyme activity:
- Temperature: Enzymes generally exhibit optimal activity within a specific temperature range. High temperatures can denature the enzyme, disrupting its three-dimensional structure and rendering it inactive. Low temperatures reduce the rate of enzyme-substrate collisions, lowering activity.
- pH: Enzymes have optimal pH ranges, and deviations from these ranges can affect the ionization state of amino acid residues within the active site, impacting substrate binding and catalytic activity.
- Substrate Concentration: Enzyme activity generally increases with increasing substrate concentration up to a point (Vmax), after which further increases have little effect.
- Enzyme Concentration: Increasing enzyme concentration directly increases the rate of the reaction, assuming substrate is not limiting.
- Inhibitors: Enzyme inhibitors are molecules that bind to enzymes and reduce their catalytic activity. Inhibitors can be competitive (competing with the substrate for the active site), non-competitive (binding to a site other than the active site), or uncompetitive (binding to the enzyme-substrate complex).
- Activators: Enzyme activators are molecules that enhance enzyme activity, often by binding to allosteric sites and inducing conformational changes that increase the affinity for the substrate or improve the catalytic efficiency.
- Allosteric Regulation: Many enzymes are subject to allosteric regulation. Allosteric effectors bind to regulatory sites distinct from the active site, inducing conformational changes that can either enhance or inhibit enzyme activity. This type of regulation allows for rapid and sensitive control of enzyme activity in response to cellular signals.
- Covalent Modification: Covalent modifications, such as phosphorylation or glycosylation, can alter enzyme activity by affecting the conformation of the enzyme or its interaction with other molecules.
Understanding how these factors influence enzyme activity is critical for comprehending how cells regulate their metabolic pathways and respond to internal and external stimuli.
Conclusion: The Importance of Enzymes in Life
Enzymes are indispensable components of all living systems. Their remarkable catalytic power, specificity, and regulation enable the vast array of biochemical reactions necessary for life. By understanding the intricacies of enzyme mechanisms, we gain a deeper appreciation for the elegance and complexity of biological processes. The information detailed above, broken down into five key steps, provides a comprehensive overview of enzyme function and highlights the critical roles they play in maintaining life. Further research into the specifics of individual enzymes continues to reveal new insights into their catalytic mechanisms and regulatory strategies, offering potential targets for therapeutic interventions and biotechnological applications.
Latest Posts
Latest Posts
-
Is Hcl And Nacl A Buffer
May 09, 2025
-
All Organic Compounds Contain The Element Carbon
May 09, 2025
-
Why Is Race A Social Construction
May 09, 2025
-
Ciclo De La Vida De Las Plantas
May 09, 2025
-
Which Description Characterizes An Ionic Bond
May 09, 2025
Related Post
Thank you for visiting our website which covers about Explain How An Enzyme Works Using The Terms From 1-5 . We hope the information provided has been useful to you. Feel free to contact us if you have any questions or need further assistance. See you next time and don't miss to bookmark.