Explain Why Electric Forces Are Essential To Forming Compounds
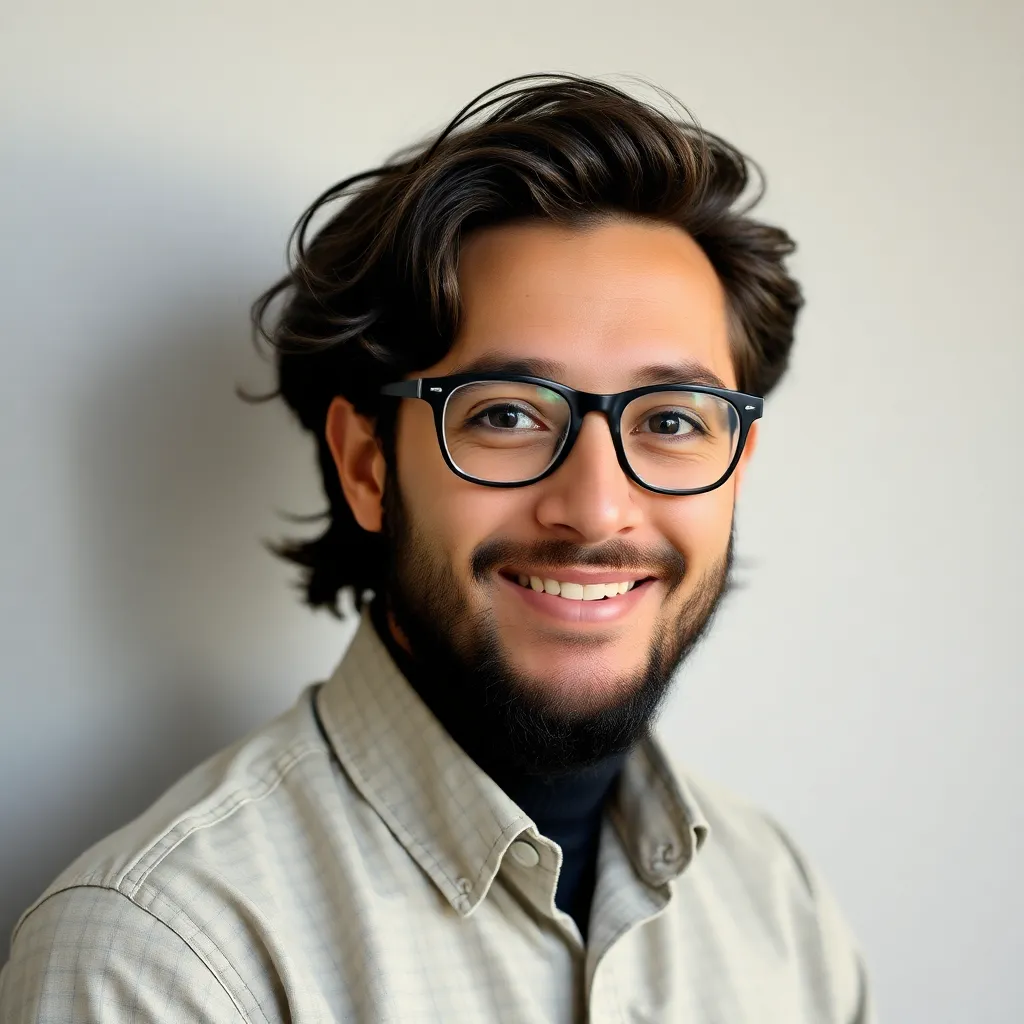
Muz Play
May 11, 2025 · 7 min read

Table of Contents
Electric Forces: The Glue of the Chemical World
The world around us is a testament to the power of chemical bonding. From the water we drink to the air we breathe, from the minerals in the earth to the complex molecules of life, compounds are the building blocks of existence. But what invisible force orchestrates the formation of these compounds? The answer lies in the realm of electromagnetism, specifically, the fundamental electric forces that attract and repel charged particles. This article delves deep into the crucial role electric forces play in compound formation, exploring various bonding types and their implications.
The Dance of Charges: Understanding Electric Forces
At the heart of chemistry lies the atom, composed of a positively charged nucleus and negatively charged electrons. These charges aren't just static labels; they exert powerful electric forces on each other and on charges in other atoms. Coulomb's Law elegantly describes this interaction: the force between two point charges is directly proportional to the product of their charges and inversely proportional to the square of the distance between them. This means stronger charges attract or repel more strongly, and the force diminishes rapidly with increasing distance.
This fundamental law provides the framework for understanding how electric forces drive chemical bonding. Electrons, being negatively charged, are naturally attracted to positively charged nuclei. This attraction, mediated by electric forces, is the driving force behind the formation of all chemical compounds. However, the specific nature of this interaction varies, leading to different types of chemical bonds.
Ionic Bonds: A Transfer of Love (Electrons)
One of the most prominent examples of electric forces in action is the ionic bond. This type of bond forms when one atom essentially donates one or more electrons to another atom. This transfer creates ions: positively charged cations (the electron donor) and negatively charged anions (the electron acceptor). The resulting electrostatic attraction between these oppositely charged ions forms the ionic bond.
Think of it like a dance: the positively charged cation is drawn to the negatively charged anion by an irresistible electric force. The stronger the charges and the closer they are, the stronger the bond. This attraction is what holds together many crystalline solids, like table salt (NaCl), where sodium (Na) loses an electron to become Na⁺ and chlorine (Cl) gains an electron to become Cl⁻. The resulting electrostatic interaction between Na⁺ and Cl⁻ ions forms the strong crystal lattice structure of salt. The properties of ionic compounds – their high melting points, brittleness, and ability to conduct electricity when molten or dissolved – are all direct consequences of these strong electrostatic interactions.
Examples of Ionic Compounds and their Electric Force Basis:
- Sodium Chloride (NaCl): The strong electrostatic attraction between Na⁺ and Cl⁻ ions leads to a high melting point and crystalline structure.
- Magnesium Oxide (MgO): Mg loses two electrons to become Mg²⁺, forming a stronger electrostatic attraction with O²⁻ than NaCl.
- Calcium Fluoride (CaF₂): One Ca²⁺ ion interacts with two F⁻ ions, demonstrating the versatility of ionic bonding.
Covalent Bonds: A Sharing Arrangement
While ionic bonds involve a complete transfer of electrons, covalent bonds arise from the sharing of electrons between atoms. This sharing isn't altruistic; it's driven by the desire of each atom to achieve a more stable electron configuration, typically a full outer electron shell (octet rule). Electric forces play a crucial role here as well. The shared electrons are attracted to the positively charged nuclei of both atoms, creating a bond that holds the atoms together.
The strength of a covalent bond depends on several factors, including the number of electrons shared (single, double, or triple bonds) and the electronegativity difference between the atoms. Electronegativity refers to an atom's ability to attract electrons in a chemical bond. If the electronegativity difference is large, the bond will have a significant polar character, meaning there's an uneven distribution of electron density. This leads to partial positive (δ⁺) and partial negative (δ⁻) charges within the molecule, creating a dipole moment. These dipoles can interact through electrostatic forces, further influencing the molecule's properties.
Examples of Covalent Compounds and Electric Force Influence:
- Water (H₂O): The oxygen atom attracts the shared electrons more strongly than the hydrogen atoms, creating polar covalent bonds and a significant dipole moment. This polarity is responsible for water's unique properties.
- Methane (CH₄): The carbon atom shares electrons with four hydrogen atoms, forming strong nonpolar covalent bonds.
- Carbon Dioxide (CO₂): Carbon forms double bonds with two oxygen atoms, resulting in a linear molecule with no net dipole moment.
Metallic Bonds: A Sea of Electrons
In metallic bonding, the valence electrons of metal atoms are delocalized, forming a "sea" of electrons that are shared among all the metal atoms. These delocalized electrons are not associated with any specific atom and are free to move throughout the metal lattice. The positive metal ions are held together by the electrostatic attraction to this "sea" of negatively charged electrons. This unique bonding explains the characteristic properties of metals, such as their high electrical and thermal conductivity, malleability, and ductility.
The strength of a metallic bond depends on the number of valence electrons and the size of the metal atoms. Metals with more valence electrons tend to form stronger metallic bonds, resulting in higher melting and boiling points. The "sea" of electrons allows for easy movement of charge, which is the reason behind the excellent conductivity of metals.
Examples of Metallic Bonds and their Electric Force Foundation:
- Copper (Cu): The delocalized valence electrons contribute to its high electrical conductivity and malleability.
- Iron (Fe): Its strong metallic bonds contribute to its high melting point and strength.
- Gold (Au): The delocalized electrons are responsible for its characteristic luster and ductility.
Hydrogen Bonding: A Special Case of Electrostatic Attraction
Hydrogen bonding is a special type of dipole-dipole interaction that occurs between molecules containing hydrogen atoms bonded to highly electronegative atoms such as oxygen, nitrogen, or fluorine. The large electronegativity difference creates a strong partial positive charge on the hydrogen atom and a strong partial negative charge on the electronegative atom. This allows for a relatively strong electrostatic attraction between the partially positive hydrogen atom of one molecule and the partially negative atom of another molecule.
Hydrogen bonds are responsible for many important biological phenomena, including the structure of DNA, the properties of water, and the stability of proteins. While weaker than ionic or covalent bonds, their collective effect can be substantial.
Intermolecular Forces: Weak, but Significant
Beyond the primary bonds discussed above, weaker intermolecular forces also contribute to the overall interactions within and between molecules. These forces, while weaker than ionic, covalent, or metallic bonds, are significant in determining the physical properties of substances like boiling points, melting points, and solubility. Examples include:
- Dipole-dipole interactions: These occur between polar molecules due to the attraction of their oppositely charged ends.
- London Dispersion Forces (LDFs): These are the weakest intermolecular forces, resulting from temporary fluctuations in electron distribution around atoms and molecules. These forces exist between all molecules, regardless of polarity.
Conclusion: The Undeniable Influence of Electric Forces
In summary, electric forces are the fundamental driving force behind the formation of all chemical compounds. From the strong electrostatic attraction in ionic bonds to the electron sharing in covalent bonds and the delocalized electron "sea" in metallic bonds, the interplay of positive and negative charges dictates the structure and properties of matter. Even weaker intermolecular forces, such as hydrogen bonding and London dispersion forces, rely on electrostatic interactions to influence the behavior of molecules. Understanding these fundamental electric forces is therefore crucial to comprehending the diverse world of chemistry and the intricate mechanisms that govern the formation and properties of all compounds around us. The seemingly simple dance of charges is ultimately the choreographer of the complex chemical world we inhabit.
Latest Posts
Related Post
Thank you for visiting our website which covers about Explain Why Electric Forces Are Essential To Forming Compounds . We hope the information provided has been useful to you. Feel free to contact us if you have any questions or need further assistance. See you next time and don't miss to bookmark.