For Every Atom Of Carbon In A Carbohydrate
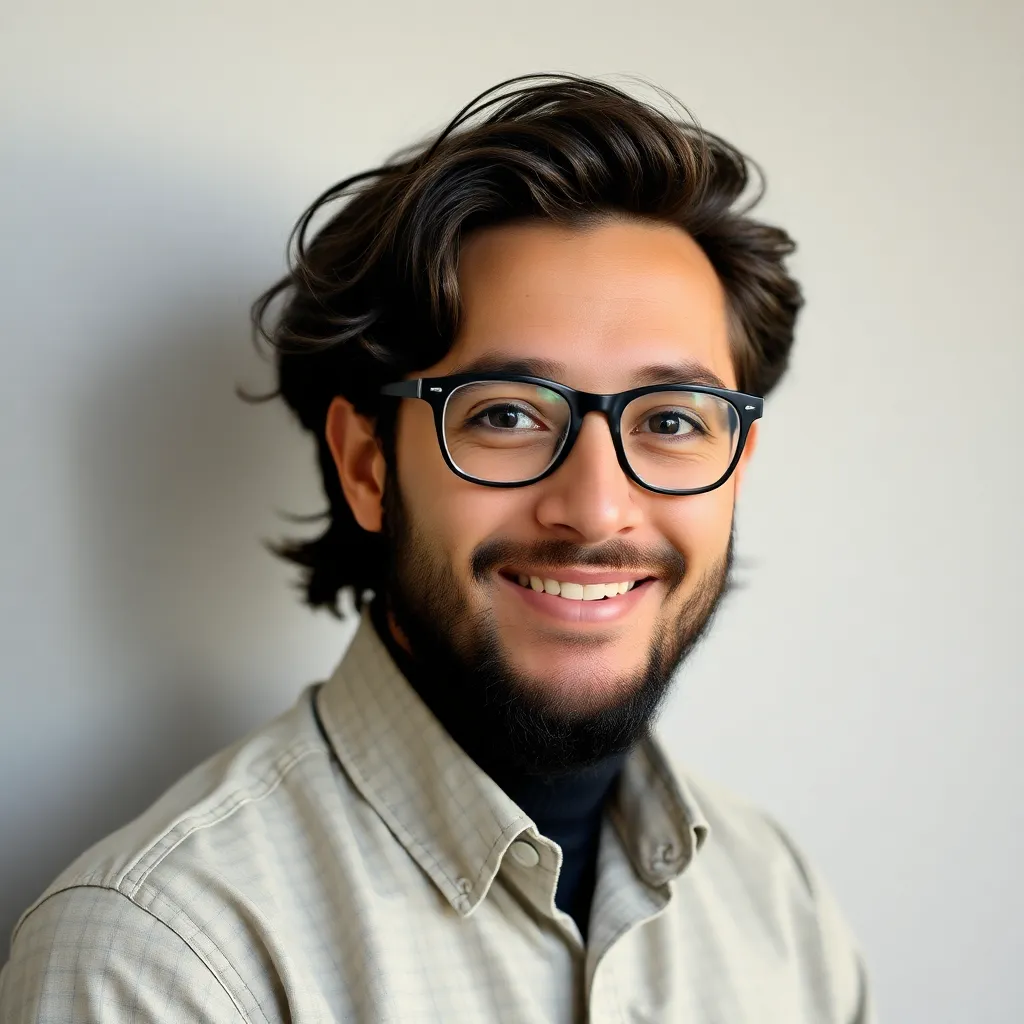
Muz Play
Apr 08, 2025 · 5 min read

Table of Contents
For Every Atom of Carbon in a Carbohydrate: A Deep Dive into the Chemistry and Biology of Sugars
Carbohydrates, the cornerstone of life, are ubiquitous in nature, forming the structural framework of plants and serving as vital energy sources for all living organisms. Understanding carbohydrates requires delving into their fundamental chemical structure, focusing on the crucial role of carbon atoms. This article will explore the intricate relationship between carbon atoms and the properties, functions, and biological significance of carbohydrates.
The Fundamental Building Block: Carbon's Central Role
At its core, a carbohydrate is a hydrated carbon, meaning its chemical formula can often be represented as (CH₂O)ₙ, where 'n' represents the number of carbon atoms. This simple formula, however, belies the incredible diversity and complexity of carbohydrate structures. For every atom of carbon in a carbohydrate, there is a specific arrangement of hydrogen and oxygen atoms that dictates its properties and function. This fundamental ratio reflects the process of photosynthesis, where plants use sunlight, water (H₂O), and carbon dioxide (CO₂) to synthesize carbohydrates.
The Carbon Backbone: Linear and Cyclic Structures
The carbon atoms in a carbohydrate form a continuous chain or backbone, which can be either linear or cyclic. Simple sugars, or monosaccharides, such as glucose and fructose, can exist in both linear and cyclic forms in equilibrium. The linear form helps to visualize the bonding arrangements, while the cyclic form is often the predominant form in aqueous solutions. This cyclization is a crucial step that leads to the formation of various isomers and the possibility of complex carbohydrate structures.
The number of carbon atoms in the monosaccharide determines its classification:
- Triose: 3 carbon atoms (e.g., glyceraldehyde)
- Tetrose: 4 carbon atoms
- Pentose: 5 carbon atoms (e.g., ribose, deoxyribose)
- Hexose: 6 carbon atoms (e.g., glucose, fructose, galactose)
- Heptose: 7 carbon atoms
Hydroxyl Groups and Isomerism: The Significance of Arrangement
Each carbon atom in a carbohydrate (with the exception of the carbonyl carbon) is bonded to a hydroxyl group (-OH). The spatial arrangement of these hydroxyl groups around the carbon atoms gives rise to different isomers. Isomers are molecules with the same chemical formula but different structural arrangements, leading to varying properties. For example, glucose, galactose, and fructose all have the chemical formula C₆H₁₂O₆ but differ in the arrangement of their hydroxyl groups, resulting in distinct biological roles.
Understanding isomerism is critical because it explains why different carbohydrates exhibit different properties:
- Structural isomers: Differ in the arrangement of atoms within the molecule.
- Stereoisomers: Have the same atom connectivity but differ in the spatial arrangement of atoms (enantiomers and diastereomers).
- Anomers: A special type of stereoisomer that differs in the configuration around the anomeric carbon (the carbon atom that forms the new chiral center during cyclization).
Building Complex Carbohydrates: Glycosidic Bonds
Monosaccharides rarely exist as isolated units in biological systems. They frequently combine to form larger molecules through glycosidic bonds. For every glycosidic bond formed, a molecule of water is removed, a process known as dehydration synthesis. This bond is formed between the anomeric carbon of one monosaccharide and a hydroxyl group on another monosaccharide.
The formation of glycosidic bonds leads to the creation of diverse carbohydrate structures:
- Disaccharides: Two monosaccharides linked by a glycosidic bond (e.g., sucrose, lactose, maltose). The properties of a disaccharide depend on the monosaccharides involved and the type of glycosidic bond linking them.
- Oligosaccharides: Short chains of monosaccharides (3-10 units). They often act as recognition signals on cell surfaces.
- Polysaccharides: Long chains of monosaccharides (hundreds or thousands of units). These are crucial for energy storage (e.g., starch, glycogen) and structural support (e.g., cellulose, chitin).
The Diversity of Polysaccharides: Function Dictated by Structure
The properties and functions of polysaccharides are intimately linked to the type of monosaccharide units, the length of the chain, the type of glycosidic bonds, and the degree of branching. For every additional monosaccharide unit added to a polysaccharide chain, the overall properties and potential functions can change significantly.
- Starch: A plant energy storage polysaccharide composed primarily of amylose (a linear chain) and amylopectin (a branched chain) – both consisting of glucose units.
- Glycogen: The animal equivalent of starch, glycogen is a highly branched glucose polymer stored in the liver and muscles. Its branching allows for rapid glucose mobilization when energy is needed.
- Cellulose: A structural polysaccharide found in plant cell walls. Its linear chains of glucose are linked by β-1,4-glycosidic bonds, forming strong and rigid fibers. Humans cannot digest cellulose due to the specific type of glycosidic bond.
- Chitin: A structural polysaccharide that forms the exoskeleton of insects and crustaceans. It is composed of N-acetylglucosamine units.
Beyond Structure: The Biological Roles of Carbohydrates
The diversity of carbohydrate structures directly correlates with their diverse biological roles. Beyond their roles as energy sources and structural components, carbohydrates play crucial roles in:
- Cell recognition and signaling: Oligosaccharides on the cell surface act as markers for cell-cell interactions, immune responses, and pathogen recognition.
- Protein glycosylation: Carbohydrates are covalently attached to proteins, altering their function, stability, and localization within the cell. This glycosylation is critical in many biological processes.
- Immune system function: Carbohydrates on the surface of pathogens trigger the immune response.
- DNA and RNA structure: Ribose (in RNA) and deoxyribose (in DNA) are pentose sugars that form the backbone of nucleic acids.
Analytical Techniques for Studying Carbohydrates
Several analytical techniques are used to study the structure and properties of carbohydrates:
- Chromatography: Techniques like thin-layer chromatography (TLC) and high-performance liquid chromatography (HPLC) are used to separate and identify different carbohydrates.
- Spectroscopy: Techniques like nuclear magnetic resonance (NMR) spectroscopy and mass spectrometry (MS) provide detailed information on the structure and composition of carbohydrates.
- X-ray crystallography: This technique is crucial in determining the three-dimensional structure of complex carbohydrates.
Conclusion: The Intricate World of Carbohydrates
For every atom of carbon in a carbohydrate, there is a story to be told. The seemingly simple chemical formula belies the incredible diversity and complexity of carbohydrate structures and functions. From the simple monosaccharides to the complex polysaccharides, these molecules are essential for life on Earth. Understanding the intricate relationship between the carbon backbone, the arrangement of hydroxyl groups, glycosidic bonds, and the resulting diverse properties is crucial for comprehending the fundamental processes of life. Further research continually reveals new aspects of carbohydrate biology, emphasizing their importance in health, disease, and biotechnology. The future of carbohydrate research holds immense potential for developing novel therapeutics and improving our understanding of biological systems.
Latest Posts
Latest Posts
-
Crossing Over Occurs Between Non Sister Chromatids
Apr 17, 2025
-
Does Fluorine Gain Or Lose Electrons
Apr 17, 2025
-
Are Chloroplast Found In Animal Cells
Apr 17, 2025
-
Pure Water Is Homogeneous Or Heterogeneous
Apr 17, 2025
-
Is Theoretical Yield The Limiting Reactant
Apr 17, 2025
Related Post
Thank you for visiting our website which covers about For Every Atom Of Carbon In A Carbohydrate . We hope the information provided has been useful to you. Feel free to contact us if you have any questions or need further assistance. See you next time and don't miss to bookmark.