Gene Expression In Bacteria Is Regulated Primarily By
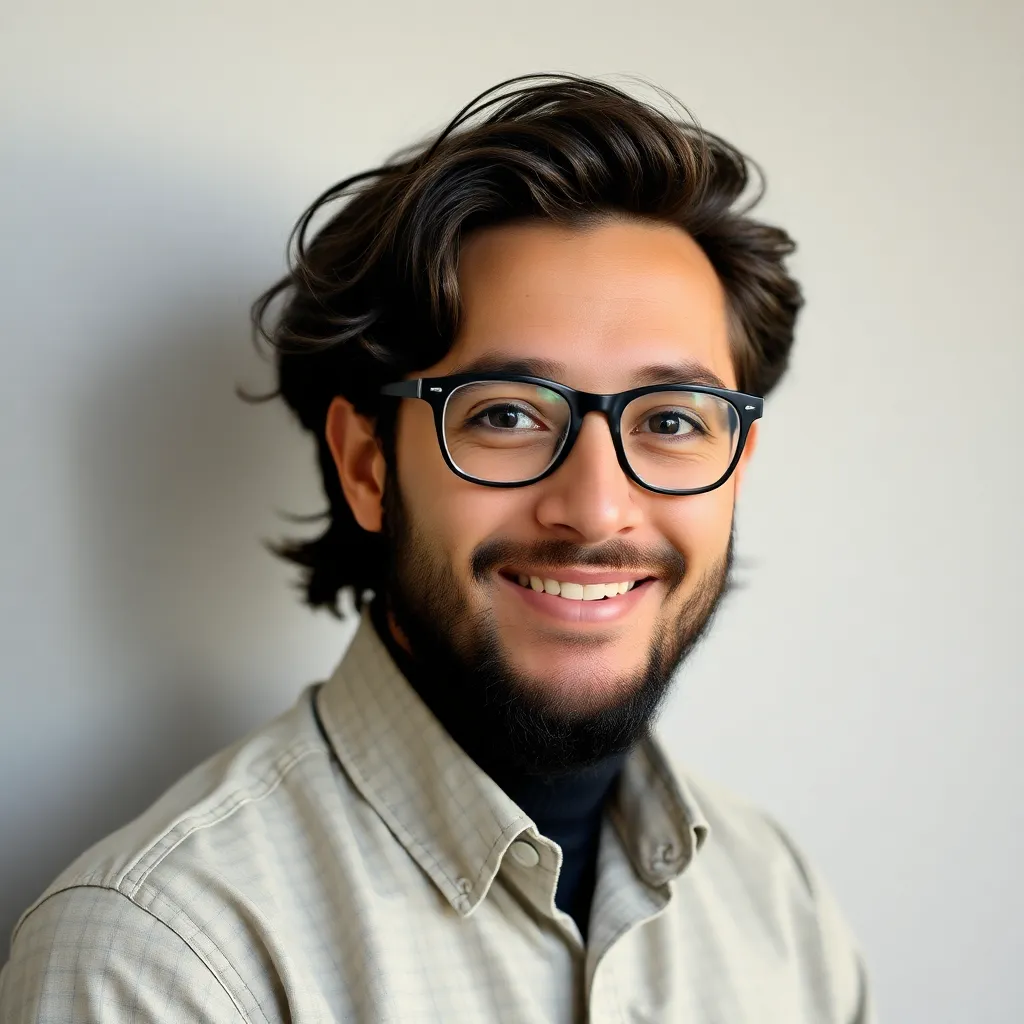
Muz Play
Apr 17, 2025 · 6 min read

Table of Contents
Gene Expression in Bacteria: A Deep Dive into Regulatory Mechanisms
Gene expression, the process by which information encoded in a gene is used to synthesize a functional gene product (typically a protein), is a tightly controlled process in all living organisms. However, in bacteria, due to their simpler structure and rapid response to environmental changes, the regulation of gene expression is particularly crucial for survival and adaptation. This article will explore the primary mechanisms by which bacteria regulate gene expression, focusing on the intricate interplay of transcriptional, translational, and post-translational control.
Transcriptional Regulation: The Master Switch
Transcriptional regulation, the control of the initiation of transcription, is the primary mechanism for regulating gene expression in bacteria. This involves controlling whether or not RNA polymerase, the enzyme responsible for transcribing DNA into RNA, binds to the promoter region of a gene. This control is largely exerted by regulatory proteins, which can either activate or repress transcription.
1. Operons: The Coordinated Units of Gene Expression
Bacterial genes involved in related metabolic pathways are often organized into operons. An operon is a cluster of genes transcribed from a single promoter, resulting in a polycistronic mRNA molecule containing the coding sequences for multiple proteins. This coordinated regulation allows for efficient and simultaneous control of genes involved in a specific metabolic process. The lac operon, responsible for lactose metabolism in E. coli, is a classic example.
2. Repressors: Turning Genes Off
Repressor proteins bind to specific DNA sequences, called operator sites, located near or overlapping the promoter region. This binding physically blocks RNA polymerase from accessing the promoter, thus preventing transcription. The lac operon's repressor, encoded by the lacI gene, is a prime example. In the absence of lactose, the repressor binds to the operator, preventing transcription of the genes required for lactose metabolism.
3. Activators: Turning Genes On
Activator proteins, on the other hand, enhance the binding of RNA polymerase to the promoter, thus increasing the rate of transcription. These proteins often bind to specific DNA sequences called activator-binding sites, located upstream of the promoter. The presence of an activator is often necessary, even for promoters that are intrinsically "weak" and poorly recognized by RNA polymerase. The catabolite activator protein (CAP), involved in the regulation of the lac operon, is a crucial example of an activator protein. CAP only binds to its DNA binding site when it is bound to cAMP, a signal molecule indicating low glucose levels.
4. Attenuation: A Fine-Tuning Mechanism
Attenuation is a regulatory mechanism that controls gene expression at the level of transcription termination. It relies on the formation of alternative secondary structures within the mRNA molecule. These structures can either allow transcription to proceed to completion or cause premature termination. A well-studied example is the trp operon, responsible for tryptophan biosynthesis in E. coli. When tryptophan levels are high, the mRNA forms a terminator hairpin structure, causing transcription to terminate prematurely. When tryptophan levels are low, a different hairpin structure forms, allowing transcription to proceed. This mechanism provides a sensitive and rapid response to fluctuations in tryptophan levels.
Translational Regulation: Control After Transcription
While transcriptional control is the primary mechanism, bacteria also employ translational regulation to fine-tune gene expression. This involves controlling the efficiency of translation initiation and elongation.
1. Riboswitches: mRNA-Based Regulation
Riboswitches are cis-acting regulatory elements located in the 5' untranslated region (UTR) of mRNA molecules. They consist of a specific RNA sequence that can directly bind to a small molecule, such as a metabolite. This binding alters the secondary structure of the mRNA, affecting the accessibility of the ribosome-binding site (RBS) and thereby influencing translation initiation. This mechanism provides a direct link between the concentration of a metabolite and the translation of genes involved in its metabolism.
2. Small RNAs (sRNAs): Post-Transcriptional Regulators
Small non-coding RNA molecules (sRNAs) play a significant role in post-transcriptional regulation. They can bind to specific mRNA target molecules, either preventing or promoting their translation. Some sRNAs bind to the RBS of their target mRNAs, hindering ribosome binding. Others can interact with other parts of the mRNA and influence its stability or translation efficiency. The expression of these sRNAs is often regulated in response to environmental cues.
3. Translational Repressors: Blocking the Ribosome
Some proteins can specifically bind to mRNA molecules and block ribosome binding to the RBS, preventing translation. These translational repressors often bind to specific sequences within the 5'UTR or the coding sequence. This mechanism offers additional layers of control over the translation of specific genes.
Post-Translational Regulation: Fine-Tuning Protein Activity
Even after a protein has been synthesized, its activity can be further regulated.
1. Proteolysis: Targeted Protein Degradation
Proteins can be selectively degraded by proteases, specific enzymes that cleave peptide bonds. This mechanism is crucial for controlling the lifespan of proteins and removing damaged or misfolded proteins. The choice of protease, the timing of protein degradation, and the specific proteins being targeted are tightly regulated.
2. Covalent Modifications: Altering Protein Function
Covalent modifications, such as phosphorylation, acetylation, or methylation, can alter the activity of proteins. These modifications often involve the addition or removal of chemical groups to specific amino acid residues, which can change the protein's conformation and its interaction with other molecules. This can activate or inhibit a protein's function, providing a rapid and reversible means of control.
Environmental Influences: The Dynamic Nature of Bacterial Gene Regulation
The regulation of gene expression in bacteria is not static; it's a highly dynamic process that responds to various environmental cues. These cues can include:
-
Nutrient availability: The presence or absence of specific nutrients, such as glucose, lactose, or amino acids, drastically influences gene expression. The lac and trp operons serve as prime examples of how nutrient availability affects gene expression.
-
Temperature: Temperature changes can trigger changes in gene expression, allowing bacteria to adapt to different temperatures. This often involves the production of heat-shock proteins that protect the cell from thermal stress.
-
Stress conditions: Exposure to stress conditions, such as osmotic shock, oxidative stress, or antibiotic treatment, can induce the expression of genes involved in stress response and survival.
-
Quorum sensing: Quorum sensing is a process whereby bacteria communicate with each other through the release and detection of signaling molecules. This allows bacteria to coordinate their gene expression based on population density.
-
Two-component regulatory systems: These systems consist of a sensor kinase protein that detects environmental stimuli and a response regulator protein that alters gene expression. These systems are ubiquitous in bacteria and allow for precise responses to diverse environmental conditions.
Conclusion: A Complex and Intertwined Regulatory Network
The regulation of gene expression in bacteria is a complex and highly interconnected process involving multiple levels of control. Transcriptional control, mediated by operons, repressors, activators, and attenuation, is the primary mechanism. However, translational and post-translational regulatory mechanisms provide further layers of control, allowing for precise adjustments in response to fluctuating environmental conditions. Understanding these regulatory mechanisms is crucial for comprehending bacterial physiology, pathogenesis, and biotechnological applications. The intricate interplay of these mechanisms ensures that bacteria can efficiently adapt to ever-changing environments and maintain optimal function. Further research into these processes will likely reveal even greater complexity and sophistication in bacterial gene regulation. This continuous exploration will contribute significantly to our understanding of microbiology and its practical applications across various fields.
Latest Posts
Latest Posts
-
Line Integral With Respect To Arc Length
Apr 19, 2025
-
What Four Bases Are Found In Rna
Apr 19, 2025
-
What Is Beyonces Song Lemonade About
Apr 19, 2025
-
Give The Major Force Between Ethanol And Water
Apr 19, 2025
-
How To Create Common Size Balance Sheet
Apr 19, 2025
Related Post
Thank you for visiting our website which covers about Gene Expression In Bacteria Is Regulated Primarily By . We hope the information provided has been useful to you. Feel free to contact us if you have any questions or need further assistance. See you next time and don't miss to bookmark.