Gene Expression Understanding The Genetic Code Answer Key
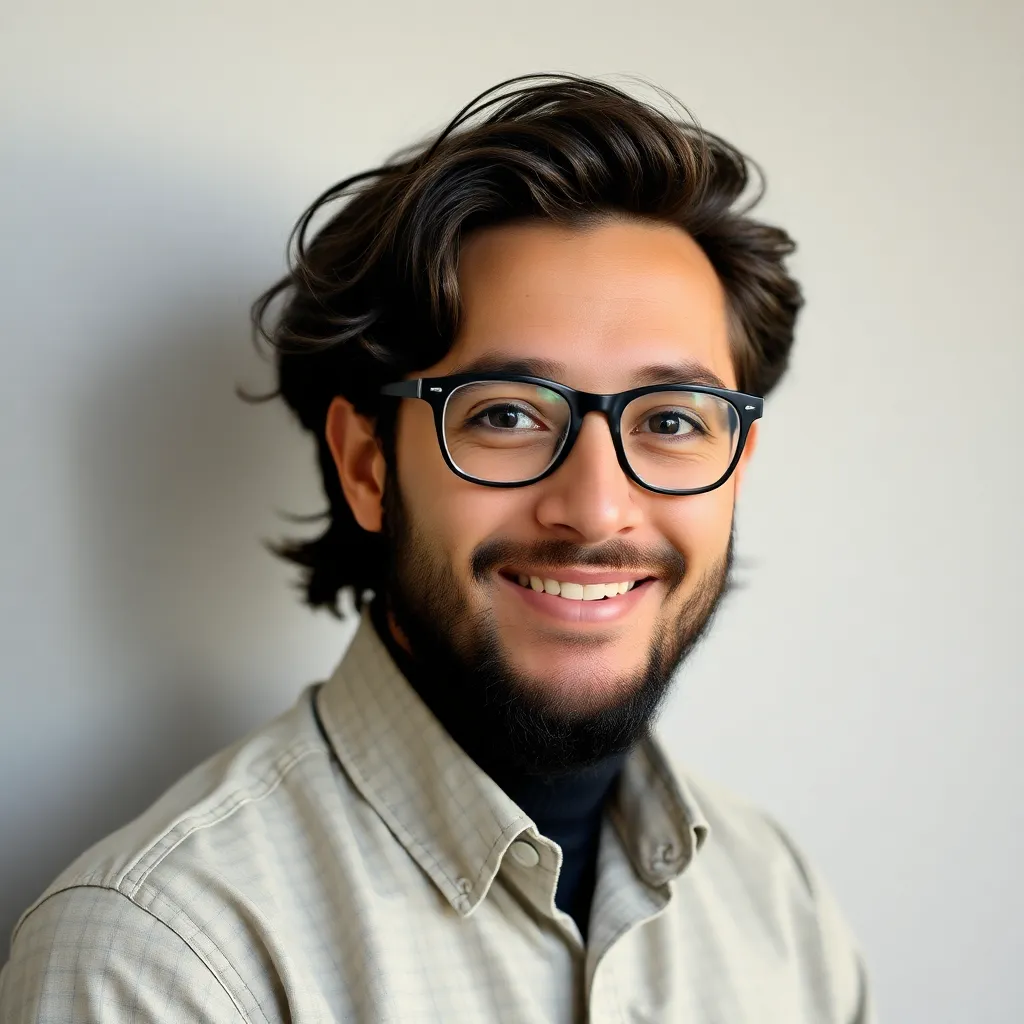
Muz Play
Apr 18, 2025 · 6 min read

Table of Contents
Gene Expression: Understanding the Genetic Code – The Comprehensive Guide
Gene expression, the process by which information from a gene is used in the synthesis of a functional gene product, be it a protein or a functional RNA molecule, is a fundamental concept in biology. Understanding this intricate process is crucial for comprehending how life functions, develops, and responds to its environment. This comprehensive guide delves into the intricacies of gene expression, exploring its various stages, regulatory mechanisms, and the implications of its dysregulation in disease.
From DNA to RNA: Transcription – The First Step in Gene Expression
The journey of gene expression begins with transcription, the process of copying a gene's DNA sequence into a messenger RNA (mRNA) molecule. This occurs in the cell's nucleus. Think of DNA as the master blueprint, and mRNA as a working copy. The enzyme responsible for this crucial step is RNA polymerase.
The Players Involved:
- DNA (Deoxyribonucleic acid): The template containing the genetic code. Its double helix structure unwinds to expose the sequence to be transcribed.
- RNA Polymerase: The enzyme that catalyzes the synthesis of mRNA. It binds to specific regions of DNA called promoters, initiating transcription.
- Promoters: Specific DNA sequences that signal the start of a gene. They act as binding sites for RNA polymerase and other transcription factors.
- Transcription Factors: Proteins that regulate the rate of transcription. They can either enhance or repress gene expression, influencing the amount of mRNA produced.
- mRNA (Messenger RNA): The RNA molecule synthesized during transcription. It carries the genetic information from the DNA to the ribosomes, where protein synthesis takes place.
The Transcription Process: Initiation, Elongation, and Termination
- Initiation: RNA polymerase binds to the promoter region of the DNA, initiating the unwinding of the DNA double helix.
- Elongation: RNA polymerase moves along the DNA template strand, synthesizing a complementary mRNA molecule. This process follows the base-pairing rules: adenine (A) pairs with uracil (U) (instead of thymine in DNA), guanine (G) pairs with cytosine (C).
- Termination: Specific DNA sequences signal the end of the gene. RNA polymerase releases the newly synthesized mRNA molecule, and the DNA double helix rewinds.
Post-Transcriptional Modifications:
The newly synthesized mRNA molecule often undergoes several modifications before it can be translated into a protein. These modifications include:
- Capping: Adding a protective cap to the 5' end of the mRNA.
- Splicing: Removing non-coding regions called introns and joining the coding regions called exons.
- Polyadenylation: Adding a poly(A) tail to the 3' end of the mRNA, which enhances stability and translation efficiency.
From RNA to Protein: Translation – The Synthesis of Life's Workhorses
Once the mature mRNA molecule is ready, it leaves the nucleus and enters the cytoplasm, where the next stage of gene expression takes place: translation. This is the process of synthesizing a protein from the mRNA template. The key players here are:
The Translation Machinery:
- mRNA (Messenger RNA): Carries the genetic code from the DNA.
- Ribosomes: Cellular structures that read the mRNA and synthesize the protein. They consist of ribosomal RNA (rRNA) and proteins.
- tRNA (Transfer RNA): Molecules that carry specific amino acids to the ribosome based on the mRNA codons. Each tRNA molecule has an anticodon that complements a specific mRNA codon.
- Amino Acids: The building blocks of proteins. Twenty different amino acids are used in protein synthesis.
- Codons: Three-nucleotide sequences on the mRNA that specify particular amino acids. The genetic code is essentially a dictionary that translates codons into amino acids.
- Anticodons: Three-nucleotide sequences on tRNA that are complementary to the mRNA codons.
The Translation Process: Initiation, Elongation, and Termination
- Initiation: The ribosome binds to the mRNA and identifies the start codon (AUG). The first tRNA molecule, carrying methionine, binds to the start codon.
- Elongation: The ribosome moves along the mRNA, reading each codon. tRNA molecules with complementary anticodons bring in the appropriate amino acids, which are linked together by peptide bonds to form a growing polypeptide chain.
- Termination: The ribosome reaches a stop codon (UAA, UAG, or UGA), which signals the end of translation. The completed polypeptide chain is released from the ribosome, and it folds into a functional protein.
Regulation of Gene Expression: A Fine-Tuned Orchestra
Gene expression is not a simple on/off switch; it's a finely tuned process regulated at multiple levels to ensure that the right proteins are produced at the right time and in the right amounts. This regulation is crucial for cellular differentiation, development, and response to environmental stimuli.
Levels of Gene Regulation:
- Transcriptional Regulation: Control of the initiation of transcription. This is the most common level of regulation, involving transcription factors, promoters, and enhancers.
- Post-Transcriptional Regulation: Control of mRNA processing, stability, and transport. This includes splicing, capping, polyadenylation, and mRNA degradation.
- Translational Regulation: Control of the initiation of translation. This can involve regulatory proteins that bind to mRNA and affect ribosome binding.
- Post-Translational Regulation: Control of protein modification, folding, and degradation. This includes phosphorylation, glycosylation, and proteasomal degradation.
Examples of Regulatory Mechanisms:
- Promoter Strength: Strong promoters lead to higher levels of transcription.
- Transcription Factor Binding: Transcription factors can either activate or repress transcription.
- Epigenetic Modifications: Chemical modifications to DNA or histones (proteins that package DNA) can affect gene expression without altering the DNA sequence. Examples include DNA methylation and histone acetylation.
- RNA Interference (RNAi): A mechanism that silences gene expression by degrading mRNA or inhibiting translation.
Dysregulation of Gene Expression and Disease
Errors in gene expression can lead to a wide range of diseases. These errors can be caused by mutations in genes, alterations in regulatory sequences, or defects in the cellular machinery involved in gene expression.
Examples of Diseases Related to Gene Expression Dysregulation:
- Cancer: Often characterized by uncontrolled cell growth due to dysregulation of genes controlling cell cycle and apoptosis (programmed cell death).
- Genetic Disorders: Many genetic disorders are caused by mutations in genes that affect protein function or expression. Examples include cystic fibrosis, sickle cell anemia, and Huntington's disease.
- Infectious Diseases: Viruses and other pathogens can alter host gene expression to promote their replication and spread.
- Neurodegenerative Diseases: Conditions like Alzheimer's and Parkinson's disease are linked to dysregulation of gene expression in neurons.
Future Directions and Research
Research into gene expression is continuously evolving, leading to advancements in our understanding of disease and the development of novel therapeutic strategies. Areas of active research include:
- CRISPR-Cas9 Gene Editing: A revolutionary technology that allows precise modification of the genome, potentially correcting faulty genes or altering gene expression.
- Epigenetics and Gene Regulation: Further investigation into the role of epigenetic modifications in disease and development.
- Single-Cell RNA Sequencing: A powerful technique that allows the analysis of gene expression in individual cells, providing insights into cellular heterogeneity and developmental processes.
- Development of Novel Therapeutics: Targeting gene expression pathways to develop new treatments for diseases.
Conclusion:
Understanding gene expression is critical for comprehending the complexities of life. From the intricate dance of transcription and translation to the finely tuned regulatory mechanisms that control gene activity, this process is central to cell function, development, and disease. Continued research in this field promises to revolutionize our understanding of health and disease, paving the way for new diagnostic tools and therapeutic strategies. The "answer key" to gene expression lies not in a single solution, but in a deep understanding of the interconnectedness of these processes and their regulation within the context of the entire organism. This guide provides a foundational understanding, prompting further exploration of this fascinating and vital area of biological study.
Latest Posts
Latest Posts
-
The Amount Of Matter In A Given Amount Of Space
Apr 19, 2025
-
Explain The Role Of A Battery In The Circuit
Apr 19, 2025
-
How To Draw A Moment Diagram
Apr 19, 2025
-
The Vitamin Required Specifically In Carbohydrate Metabolism Is
Apr 19, 2025
-
How To Find The Rectangular Coordinates
Apr 19, 2025
Related Post
Thank you for visiting our website which covers about Gene Expression Understanding The Genetic Code Answer Key . We hope the information provided has been useful to you. Feel free to contact us if you have any questions or need further assistance. See you next time and don't miss to bookmark.