Glycolysis Can Occur In Either Aerobic Or Anaerobic Conditions.
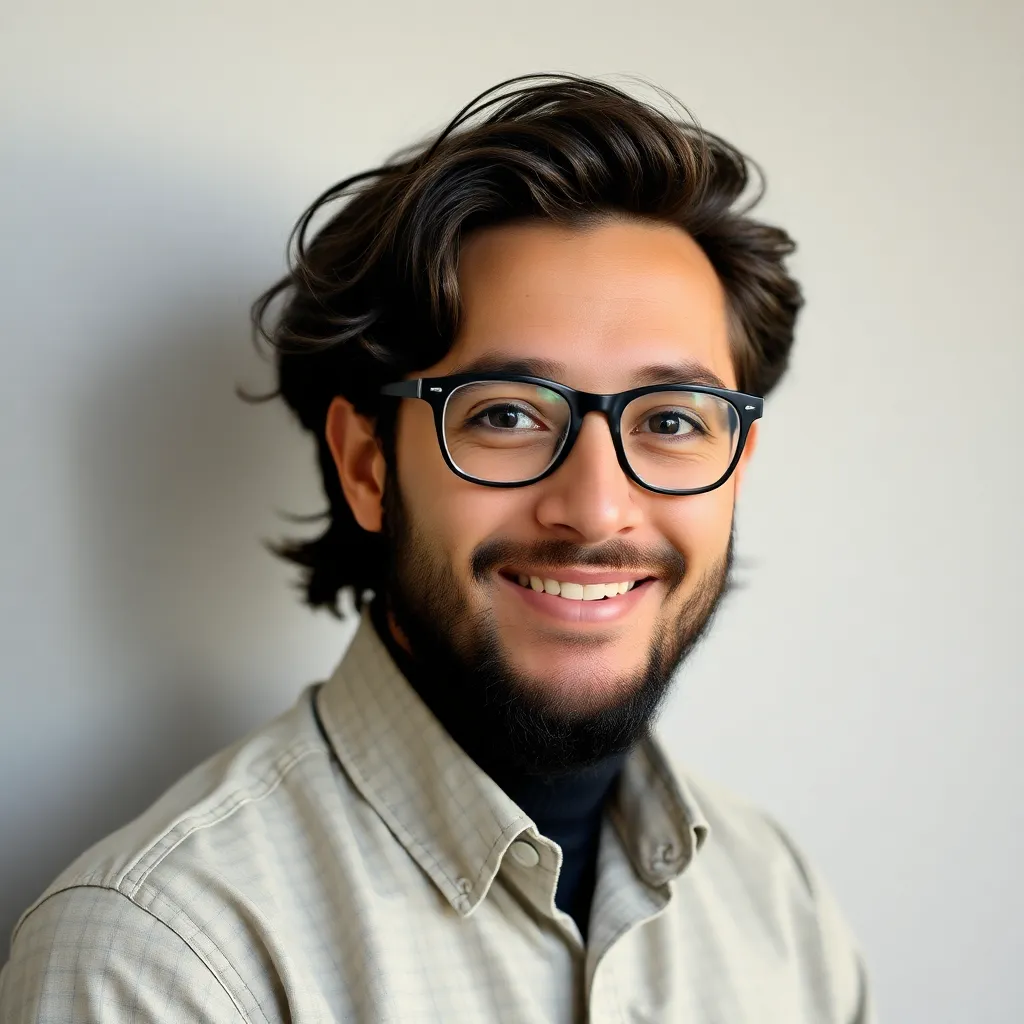
Muz Play
Apr 24, 2025 · 6 min read

Table of Contents
Glycolysis: The Universal Energy Pathway – Aerobic and Anaerobic Operation
Glycolysis, the metabolic pathway responsible for the initial breakdown of glucose, stands as a cornerstone of cellular energy production. Remarkably, this process can occur under both aerobic (oxygen-rich) and anaerobic (oxygen-deficient) conditions, highlighting its fundamental importance across a vast spectrum of life. Understanding the intricacies of glycolysis under both scenarios is key to comprehending cellular respiration, fermentation, and the overall energy balance within living organisms. This comprehensive exploration will delve into the details of glycolysis, focusing on its remarkable adaptability to diverse environmental conditions.
The Core Steps of Glycolysis: A Universal Process
Before delving into the aerobic and anaerobic variations, let's establish a firm understanding of the fundamental steps of glycolysis. This ten-step pathway, occurring in the cytoplasm of the cell, involves a series of enzyme-catalyzed reactions that convert a single molecule of glucose (a six-carbon sugar) into two molecules of pyruvate (a three-carbon compound). This process can be broadly divided into two phases: the energy-investment phase and the energy-payoff phase.
The Energy-Investment Phase: Priming the Pump
This initial phase requires an investment of energy in the form of two ATP molecules. These ATP molecules are utilized to phosphorylate glucose, making it more reactive and trapping it within the cell. Key steps in this phase include:
- Hexokinase: This enzyme catalyzes the phosphorylation of glucose to glucose-6-phosphate, using ATP. This step is essentially irreversible under cellular conditions.
- Phosphoglucose Isomerase: This enzyme converts glucose-6-phosphate to fructose-6-phosphate, an isomerization reaction.
- Phosphofructokinase (PFK): This crucial regulatory enzyme catalyzes the phosphorylation of fructose-6-phosphate to fructose-1,6-bisphosphate, consuming another ATP molecule. This step is another highly regulated and irreversible step, acting as a major control point for glycolysis.
- Aldolase: This enzyme cleaves fructose-1,6-bisphosphate into two three-carbon molecules: glyceraldehyde-3-phosphate (G3P) and dihydroxyacetone phosphate (DHAP).
The Energy-Payoff Phase: Harvesting the Energy
The second phase focuses on extracting energy from the two three-carbon molecules generated in the first phase. This phase yields a net gain of ATP and NADH, a crucial electron carrier. Key steps include:
- Triose Phosphate Isomerase: DHAP is readily isomerized to G3P, ensuring that both three-carbon molecules contribute to the subsequent steps.
- Glyceraldehyde-3-phosphate Dehydrogenase: This enzyme catalyzes the oxidation of G3P, generating NADH and a high-energy phosphate intermediate, 1,3-bisphosphoglycerate.
- Phosphoglycerate Kinase: This enzyme transfers a phosphate group from 1,3-bisphosphoglycerate to ADP, generating ATP through substrate-level phosphorylation. This step is a crucial source of ATP production.
- Phosphoglycerate Mutase: This enzyme relocates the phosphate group within the 3-phosphoglycerate molecule, converting it to 2-phosphoglycerate.
- Enolase: This enzyme dehydrates 2-phosphoglycerate, forming phosphoenolpyruvate (PEP), a high-energy compound.
- Pyruvate Kinase: This enzyme catalyzes the transfer of a phosphate group from PEP to ADP, generating another molecule of ATP through substrate-level phosphorylation and yielding pyruvate.
Glycolysis Under Aerobic Conditions: The Aerobic Fate of Pyruvate
In the presence of oxygen, pyruvate, the end product of glycolysis, doesn't accumulate. Instead, it enters the mitochondria, the powerhouse of the cell, to undergo further oxidation. This is where the significant energy yield from glucose is realized. The process continues through the following steps:
- Pyruvate Oxidation: Pyruvate is converted to acetyl-CoA, a two-carbon molecule, releasing CO2 and generating NADH. This step occurs within the mitochondrial matrix.
- Krebs Cycle (Citric Acid Cycle): Acetyl-CoA enters the Krebs cycle, a series of reactions that further oxidizes the carbon atoms, releasing more CO2 and generating ATP, NADH, and FADH2 (another electron carrier).
- Oxidative Phosphorylation: NADH and FADH2 donate their electrons to the electron transport chain (ETC), a series of protein complexes embedded in the inner mitochondrial membrane. The flow of electrons down the ETC drives proton pumping, creating a proton gradient across the membrane. This gradient is then harnessed by ATP synthase to generate a significant amount of ATP through chemiosmosis. This is the most efficient way for cells to produce ATP. Oxygen acts as the final electron acceptor in the ETC, forming water.
This complete aerobic pathway, encompassing glycolysis, pyruvate oxidation, the Krebs cycle, and oxidative phosphorylation, extracts a maximum of 36-38 ATP molecules from a single glucose molecule – a substantially higher yield than glycolysis alone.
Glycolysis Under Anaerobic Conditions: Fermentation – A Backup Plan
In the absence of oxygen, the fate of pyruvate is drastically altered. The electron transport chain cannot function effectively without oxygen as the final electron acceptor, leading to a buildup of NADH. To regenerate NAD+, which is essential for glycolysis to continue, cells resort to fermentation.
Fermentation is a metabolic process that regenerates NAD+ from NADH, allowing glycolysis to proceed even without oxygen. Two common types of fermentation are:
Lactic Acid Fermentation: Muscles Under Strain
In lactic acid fermentation, pyruvate is directly reduced to lactate, regenerating NAD+. This process is prominent in muscle cells during strenuous exercise when oxygen supply becomes limited. The accumulation of lactate contributes to muscle fatigue and soreness. Lactic acid fermentation is also utilized by some bacteria and fungi.
Alcoholic Fermentation: The Basis of Brewing and Baking
In alcoholic fermentation, pyruvate is first decarboxylated to acetaldehyde, releasing CO2. Acetaldehyde is then reduced to ethanol, regenerating NAD+. This process is used by yeasts and some bacteria, and it's the basis for the production of alcoholic beverages and leavened bread. The CO2 produced during alcoholic fermentation contributes to the rise of bread dough.
While fermentation regenerates NAD+, it's far less efficient than aerobic respiration in terms of ATP production. Glycolysis under anaerobic conditions yields only 2 ATP molecules per glucose molecule, significantly less than the 36-38 ATP molecules produced during aerobic respiration. This explains why organisms relying primarily on anaerobic metabolism generally grow and reproduce more slowly.
The Regulation of Glycolysis: A Fine-Tuned System
The rate of glycolysis is tightly regulated to meet the cell's energy demands. Several key enzymes are subject to allosteric regulation, meaning their activity is modulated by the binding of small molecules.
- Phosphofructokinase (PFK): This enzyme is a major regulatory point in glycolysis. ATP and citrate (an intermediate in the Krebs cycle) inhibit PFK, slowing down glycolysis when energy levels are high. AMP and ADP activate PFK, stimulating glycolysis when energy levels are low.
- Hexokinase: This enzyme is inhibited by its product, glucose-6-phosphate, preventing excessive glucose phosphorylation when glucose-6-phosphate levels are high.
- Pyruvate Kinase: This enzyme is also regulated allosterically, with ATP and alanine (an amino acid) acting as inhibitors and fructose-1,6-bisphosphate acting as an activator.
Hormonal regulation also plays a significant role in controlling glycolysis. Insulin, for example, stimulates glycolysis in the liver and muscle cells, while glucagon has the opposite effect.
Glycolysis in Different Organisms: A Universal Process with Variations
While the basic steps of glycolysis are conserved across a wide range of organisms, minor variations exist. For instance, some organisms use different enzymes or slightly altered pathways to achieve the same overall result. These variations often reflect adaptations to specific environmental conditions or metabolic needs.
Conclusion: A Remarkable Metabolic Pathway
Glycolysis's ability to operate under both aerobic and anaerobic conditions showcases its versatility and fundamental role in cellular metabolism. Whether it fuels the intense activity of muscle cells or the slow fermentation process of yeasts, glycolysis remains a crucial pathway providing the foundational energy required for life's processes. The intricate regulation of glycolysis, coupled with its adaptability to different oxygen levels, highlights the sophisticated control mechanisms that govern cellular energy production. Further research continues to unravel the intricacies of this remarkable metabolic pathway and its implications for various biological processes and disease states.
Latest Posts
Latest Posts
-
A Bronsted Lowry Base Is Defined As A Substance That
Apr 24, 2025
-
Describe The Four Stages Of The Demographic Transition
Apr 24, 2025
-
Which Of The Following Are Types Of Chromosomal Alterations
Apr 24, 2025
-
What Level Of Measurement Is Temperature
Apr 24, 2025
-
Why Do Animal Cells Lack Chloroplasts
Apr 24, 2025
Related Post
Thank you for visiting our website which covers about Glycolysis Can Occur In Either Aerobic Or Anaerobic Conditions. . We hope the information provided has been useful to you. Feel free to contact us if you have any questions or need further assistance. See you next time and don't miss to bookmark.