How Do Lone Pairs Affect Hybridization
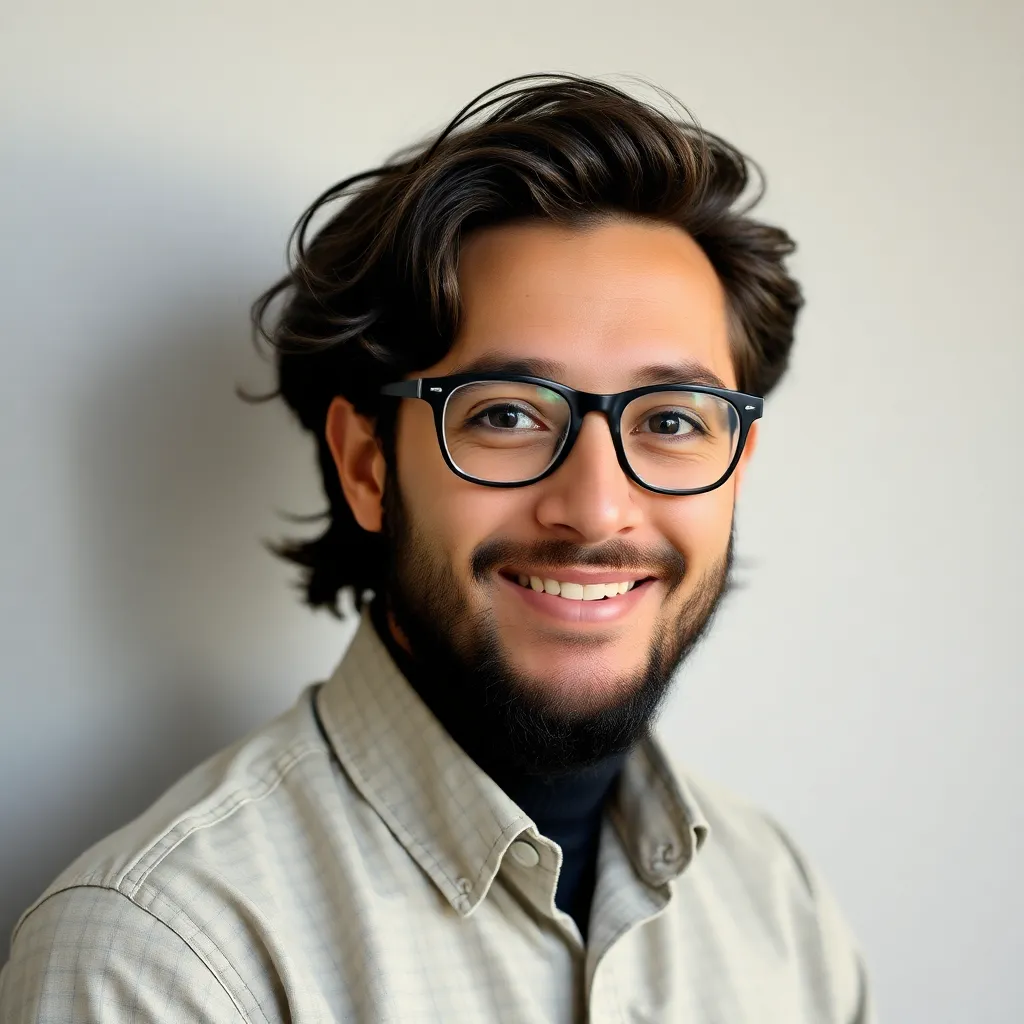
Muz Play
Apr 23, 2025 · 5 min read

Table of Contents
How Do Lone Pairs Affect Hybridization? A Comprehensive Guide
Understanding hybridization is crucial for predicting molecular geometry and properties. While the basic concept focuses on the mixing of atomic orbitals to form hybrid orbitals, the presence of lone pairs significantly influences the final shape and characteristics of a molecule. This article delves deep into the effects of lone pairs on hybridization, explaining the concepts clearly and comprehensively.
Understanding Hybridization: A Quick Recap
Hybridization is a theoretical concept explaining the bonding in molecules where atomic orbitals combine to form new hybrid orbitals with different shapes and energies than the original atomic orbitals. The most common types are:
- sp: One s orbital and one p orbital combine to form two sp hybrid orbitals, arranged linearly (180° bond angle).
- sp²: One s orbital and two p orbitals combine to form three sp² hybrid orbitals, arranged trigonally planar (120° bond angle).
- sp³: One s orbital and three p orbitals combine to form four sp³ hybrid orbitals, arranged tetrahedrally (109.5° bond angle).
These hybrid orbitals are then used to form sigma (σ) bonds with other atoms. The remaining unhybridized p orbitals can participate in the formation of pi (π) bonds.
The Influence of Lone Pairs on Hybridization
Lone pairs, or non-bonding pairs of electrons, occupy hybrid orbitals just like bonding pairs. However, their influence on molecular geometry is different. They exert a stronger repulsive force than bonding pairs, leading to deviations from ideal bond angles predicted by simple hybridization models.
Why are Lone Pair Repulsions Stronger?
Lone pairs are not constrained by being shared between two nuclei like bonding pairs. This means they occupy a larger volume of space around the central atom, leading to greater repulsion with other electron pairs (both bonding and lone pairs).
Impact on Molecular Geometry: Case Studies
Let's examine how lone pairs affect the molecular geometry of various molecules, using the VSEPR (Valence Shell Electron Pair Repulsion) theory as a guiding principle. VSEPR theory predicts that electron pairs around a central atom will arrange themselves to minimize repulsion.
sp³ Hybridization Examples
1. Methane (CH₄): Carbon in methane uses sp³ hybridization, forming four σ bonds with four hydrogen atoms. There are no lone pairs on the carbon atom. The resulting molecular geometry is a perfect tetrahedron with a bond angle of 109.5°.
2. Ammonia (NH₃): Nitrogen in ammonia also uses sp³ hybridization. However, it forms three σ bonds with three hydrogen atoms and has one lone pair of electrons. The lone pair occupies one of the four sp³ hybrid orbitals. The repulsion from the lone pair pushes the bonding pairs slightly closer together, resulting in a trigonal pyramidal geometry with a bond angle slightly less than 109.5° (approximately 107°).
3. Water (H₂O): Oxygen in water uses sp³ hybridization, forming two σ bonds with two hydrogen atoms and possessing two lone pairs. The two lone pairs exert a stronger repulsive force than the bonding pairs, causing a significant reduction in the bond angle. The resulting molecular geometry is bent or V-shaped, with a bond angle of approximately 104.5°.
sp² Hybridization Examples
1. Boron Trifluoride (BF₃): Boron uses sp² hybridization, forming three σ bonds with three fluorine atoms. There are no lone pairs on the boron atom. The geometry is trigonal planar with a bond angle of 120°.
2. Sulfur Dioxide (SO₂): Sulfur in SO₂ uses sp² hybridization, forming two σ bonds with two oxygen atoms and having one lone pair. The lone pair reduces the bond angle to less than 120°, resulting in a bent molecular geometry.
sp Hybridization Examples
1. Beryllium Dichloride (BeCl₂): Beryllium in BeCl₂ uses sp hybridization, forming two σ bonds with two chlorine atoms. There are no lone pairs on the beryllium atom. The resulting molecular geometry is linear with a bond angle of 180°.
2. Carbon Dioxide (CO₂): While carbon in CO₂ forms double bonds with oxygen, the sigma bonds are formed from sp hybridized orbitals. No lone pairs are present on the carbon atom, maintaining the linear geometry with a bond angle of 180°.
Predicting Molecular Geometry with Lone Pairs
To predict the molecular geometry when lone pairs are present, we can use the AXE notation:
- A: Represents the central atom.
- X: Represents the number of bonding pairs of electrons.
- E: Represents the number of lone pairs of electrons.
For example:
- NH₃ (AX₃E): A (Nitrogen) is bonded to three X (Hydrogen) atoms and has one E (lone pair).
- H₂O (AX₂E₂): A (Oxygen) is bonded to two X (Hydrogen) atoms and has two E (lone pairs).
Using this notation, and understanding the relative repulsive forces, we can accurately predict the molecular geometry.
Lone Pairs and Hybridization: Beyond the Basics
The impact of lone pairs extends beyond simple geometry predictions. They influence:
- Bond Strength: Lone pair electrons can participate in resonance structures, affecting bond strength and length.
- Polarity: Lone pairs contribute to the overall dipole moment of a molecule, influencing its polarity and interactions with other molecules.
- Reactivity: Lone pairs often act as nucleophiles (electron donors), participating in chemical reactions.
- Spectroscopic Properties: The presence of lone pairs affects various spectroscopic properties such as infrared (IR) and nuclear magnetic resonance (NMR) spectra.
Advanced Considerations and Exceptions
While the concepts discussed provide a strong foundation, certain complexities arise in more advanced cases:
- Hypervalency: Some molecules exhibit expanded octets, possessing more than eight valence electrons around the central atom. This often involves the participation of d orbitals in hybridization, creating complexities beyond sp, sp², and sp³.
- d-orbital participation: Transition metal complexes often involve d-orbital participation in hybridization, further complicating geometry predictions.
- Resonance structures: Resonance significantly impacts the distribution of electron density, which in turn can influence the overall geometry and bond characteristics.
Conclusion
Lone pairs significantly affect hybridization by influencing the molecular geometry and various other molecular properties. Understanding their impact is critical for accurate predictions and the interpretation of chemical behavior. While simple models provide a good starting point, more advanced considerations are needed for complex molecules and situations. This comprehensive guide has aimed to provide a solid understanding of how lone pairs impact hybridization, equipping readers with knowledge to approach diverse chemical scenarios. By mastering these concepts, one can better understand and predict the behavior of molecules in various chemical environments. Remember to utilize the VSEPR theory and AXE notation to help visualize and predict molecular shapes accurately. Through continued learning and exploration, a deeper understanding of this fundamental aspect of chemistry is achievable.
Latest Posts
Latest Posts
-
Graph The Complex Conjugate Of The Given Number
Apr 23, 2025
-
Trust Thyself Every Heart Vibrates To That Iron String Meaning
Apr 23, 2025
-
Compare And Contrast Intermolecular Forces And Describe Intramolecular Forces
Apr 23, 2025
-
Photosystem I And Photosystem Ii Are Part Of
Apr 23, 2025
-
How Many Valence Electrons Are In Group 15
Apr 23, 2025
Related Post
Thank you for visiting our website which covers about How Do Lone Pairs Affect Hybridization . We hope the information provided has been useful to you. Feel free to contact us if you have any questions or need further assistance. See you next time and don't miss to bookmark.