How Do Single-celled Decomposers Get Energy
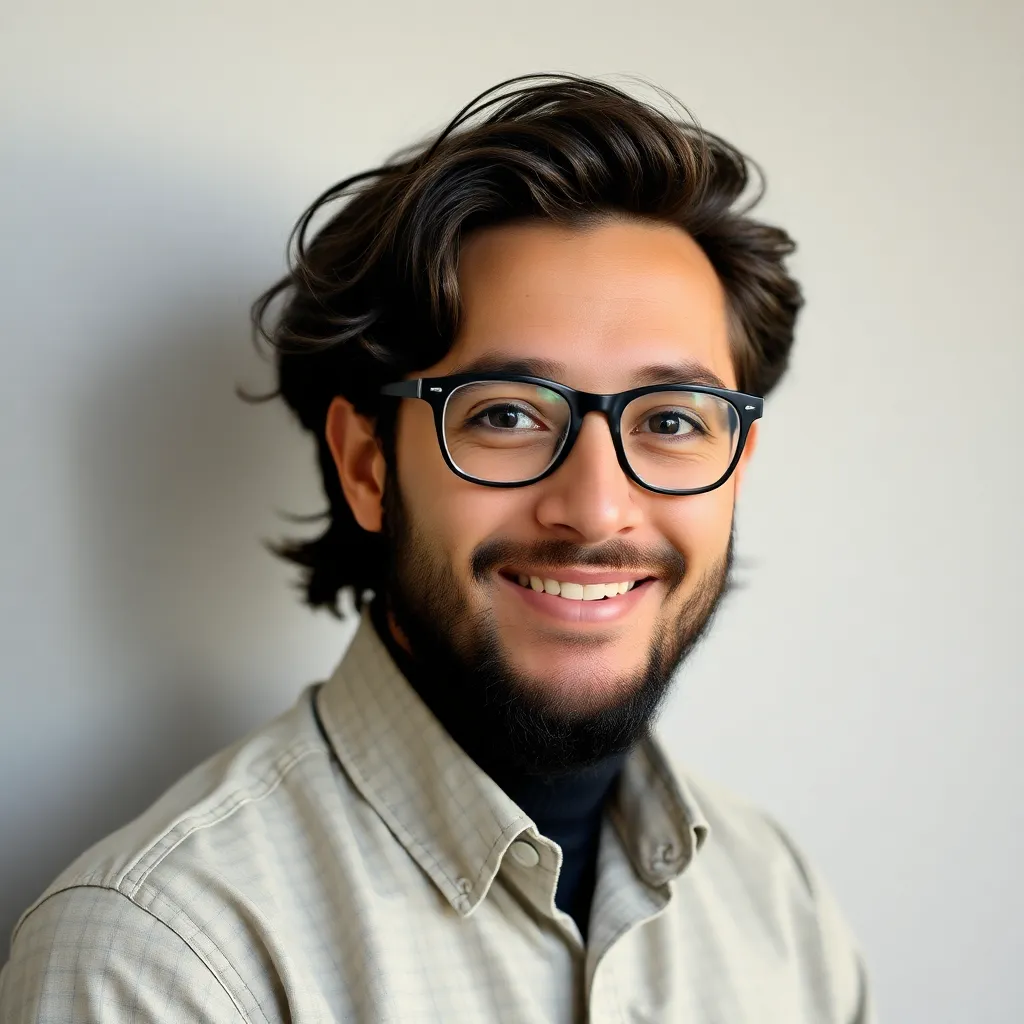
Muz Play
May 11, 2025 · 6 min read
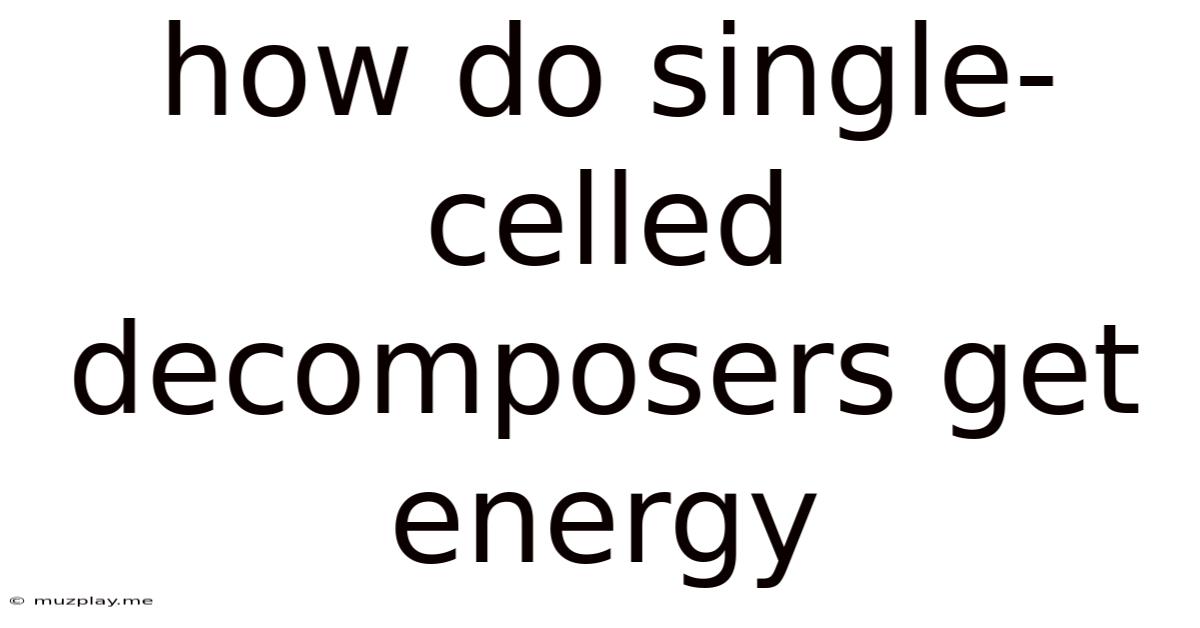
Table of Contents
How Do Single-Celled Decomposers Get Energy?
Single-celled decomposers, the microscopic heroes of the ecosystem, play a vital role in the cycle of life and death. These tiny organisms, primarily bacteria and archaea, are responsible for breaking down dead organic matter, recycling nutrients, and ensuring the continued flow of energy through various food webs. But how do these seemingly simple life forms actually obtain the energy they need to survive and thrive? The answer lies in their diverse metabolic strategies, which involve a fascinating array of biochemical processes. This comprehensive guide delves into the intricate world of single-celled decomposers, exploring the various ways they harvest energy from their environment.
The Fundamental Role of Decomposition
Before diving into the specifics of energy acquisition, it's crucial to understand the overarching importance of decomposition. Decomposers, also known as saprotrophs, are heterotrophs, meaning they cannot produce their own food through photosynthesis like plants. Instead, they obtain their energy and nutrients by breaking down complex organic molecules found in dead plants, animals, and other organic waste. This process is vital because it:
- Recycles nutrients: Decomposers release essential nutrients like nitrogen, phosphorus, and carbon back into the environment, making them available for plants and other organisms. Without them, these nutrients would remain locked within dead matter, hindering the growth and survival of other life forms.
- Prevents the buildup of organic waste: Imagine a world without decomposers – it would quickly become overwhelmed with decaying organic matter. Decomposers prevent this buildup, maintaining ecosystem balance and preventing the spread of disease.
- Supports food webs: The energy released during decomposition supports various food webs. Smaller organisms feed on decomposers, and those organisms, in turn, become food for larger animals. This intricate network ensures the efficient transfer of energy through the ecosystem.
Diverse Metabolic Pathways for Energy Harvesting
Single-celled decomposers employ a stunning variety of metabolic pathways to break down organic matter and extract energy. These pathways can be broadly categorized based on the terminal electron acceptor used in their respiration processes:
1. Aerobic Respiration: Oxygen as the Final Electron Acceptor
Aerobic respiration is the most efficient energy-harvesting pathway, utilized by many bacteria and archaea. This process requires oxygen as the final electron acceptor in the electron transport chain. The breakdown of organic molecules, such as carbohydrates, lipids, and proteins, generates ATP (adenosine triphosphate), the primary energy currency of cells.
- Glycolysis: This initial stage occurs in the cytoplasm and breaks down glucose into pyruvate, yielding a small amount of ATP.
- Krebs Cycle (Citric Acid Cycle): Pyruvate is further oxidized in the Krebs cycle, producing more ATP, NADH, and FADH2 (electron carriers).
- Electron Transport Chain (ETC): Electrons from NADH and FADH2 are passed along a series of protein complexes embedded in the cell membrane. This process generates a proton gradient, which drives ATP synthesis through chemiosmosis. Oxygen acts as the final electron acceptor, forming water.
Aerobic respiration is highly efficient, yielding a substantial amount of ATP per glucose molecule. However, it requires the presence of oxygen, limiting its use in oxygen-poor environments.
2. Anaerobic Respiration: Alternatives to Oxygen
In environments lacking oxygen, many decomposers utilize anaerobic respiration. This process uses alternative electron acceptors such as nitrate (NO₃⁻), sulfate (SO₄²⁻), or carbon dioxide (CO₂). While less efficient than aerobic respiration, anaerobic respiration still allows these organisms to extract energy from organic matter.
- Nitrate Reduction (Denitrification): Certain bacteria utilize nitrate as the final electron acceptor, converting it to nitrite (NO₂⁻), nitric oxide (NO), nitrous oxide (N₂O), and ultimately nitrogen gas (N₂). This process is crucial in the nitrogen cycle, releasing nitrogen back into the atmosphere.
- Sulfate Reduction: Sulfate-reducing bacteria use sulfate as the final electron acceptor, producing hydrogen sulfide (H₂S), a gas with a characteristic rotten-egg smell. This process is important in sulfur cycling.
- Methanogenesis: Archaea known as methanogens use carbon dioxide as the final electron acceptor, producing methane (CH₄), a potent greenhouse gas. Methanogenesis is prevalent in anaerobic environments like swamps, marshes, and the digestive tracts of animals.
3. Fermentation: ATP Production Without Electron Transport Chains
Fermentation is an anaerobic process that does not involve an electron transport chain. Instead, it relies on substrate-level phosphorylation to generate ATP. This process is less efficient than respiration, yielding far less ATP per glucose molecule. Various types of fermentation exist, depending on the end products produced:
- Lactic Acid Fermentation: Bacteria like Lactobacillus convert pyruvate to lactic acid. This process is used in the production of yogurt and sauerkraut.
- Alcoholic Fermentation: Yeasts convert pyruvate to ethanol and carbon dioxide. This is responsible for the production of alcoholic beverages and bread.
- Other fermentation pathways: Many other fermentation pathways exist, resulting in various end products, including acetic acid, propionic acid, and butyric acid.
Specific Examples of Single-celled Decomposers and their Energy Strategies
Let's examine some specific examples of single-celled decomposers and the energy strategies they employ:
- Escherichia coli (E. coli): This bacterium is a facultative anaerobe, meaning it can switch between aerobic and anaerobic respiration depending on the availability of oxygen. In the presence of oxygen, it uses aerobic respiration; in its absence, it resorts to fermentation.
- Pseudomonas aeruginosa: This bacterium is a versatile decomposer that can utilize a wide range of organic molecules as energy sources. It primarily utilizes aerobic respiration but can also utilize anaerobic respiration under anaerobic conditions.
- Clostridium spp.: These bacteria are obligate anaerobes, meaning they cannot survive in the presence of oxygen. They rely on fermentation or anaerobic respiration to obtain energy. Several species are involved in breaking down cellulose and other complex carbohydrates.
- Methanogens (Archaea): These unique microorganisms exclusively utilize methanogenesis to obtain energy, playing a crucial role in the global carbon cycle. They are vital in anaerobic environments like wetlands and ruminant animal digestive tracts.
Factors Influencing Energy Acquisition in Decomposers
Several factors influence the ability of single-celled decomposers to acquire energy:
- Nutrient availability: The abundance and type of organic matter available significantly impact the growth and activity of decomposers.
- Oxygen availability: The presence or absence of oxygen dictates which metabolic pathways are utilized.
- Temperature: Temperature affects enzyme activity and, consequently, the rate of decomposition.
- pH: The acidity or alkalinity of the environment can influence the activity of decomposers.
- Competition: Decomposers often compete with each other for resources, influencing their growth and energy acquisition.
Conclusion: The Unsung Heroes of Energy Flow
Single-celled decomposers are indispensable to the functioning of ecosystems. Their ability to harness energy from dead organic matter through diverse metabolic pathways is essential for nutrient cycling, waste removal, and the continued flow of energy through food webs. Understanding their intricate energy acquisition strategies is critical for appreciating their crucial role in maintaining the health and balance of our planet. Further research continues to uncover the remarkable diversity and complexity of these microscopic powerhouses, highlighting the ongoing importance of their study in fields ranging from environmental science to biotechnology. Their efficiency and adaptability underscore their essential role in the intricate tapestry of life.
Latest Posts
Latest Posts
-
Period Of A Mass Spring System
May 12, 2025
-
On The Weak Acid Strong Base Titration Curve Label
May 12, 2025
-
How To Find Vertical Asymptotes Of Trig Functions
May 12, 2025
-
Is Compare And Contrast A Literary Device
May 12, 2025
-
Which Of The Following Is An Example Of A Heterotroph
May 12, 2025
Related Post
Thank you for visiting our website which covers about How Do Single-celled Decomposers Get Energy . We hope the information provided has been useful to you. Feel free to contact us if you have any questions or need further assistance. See you next time and don't miss to bookmark.