How Does An Enzyme Affect A Chemical Reaction
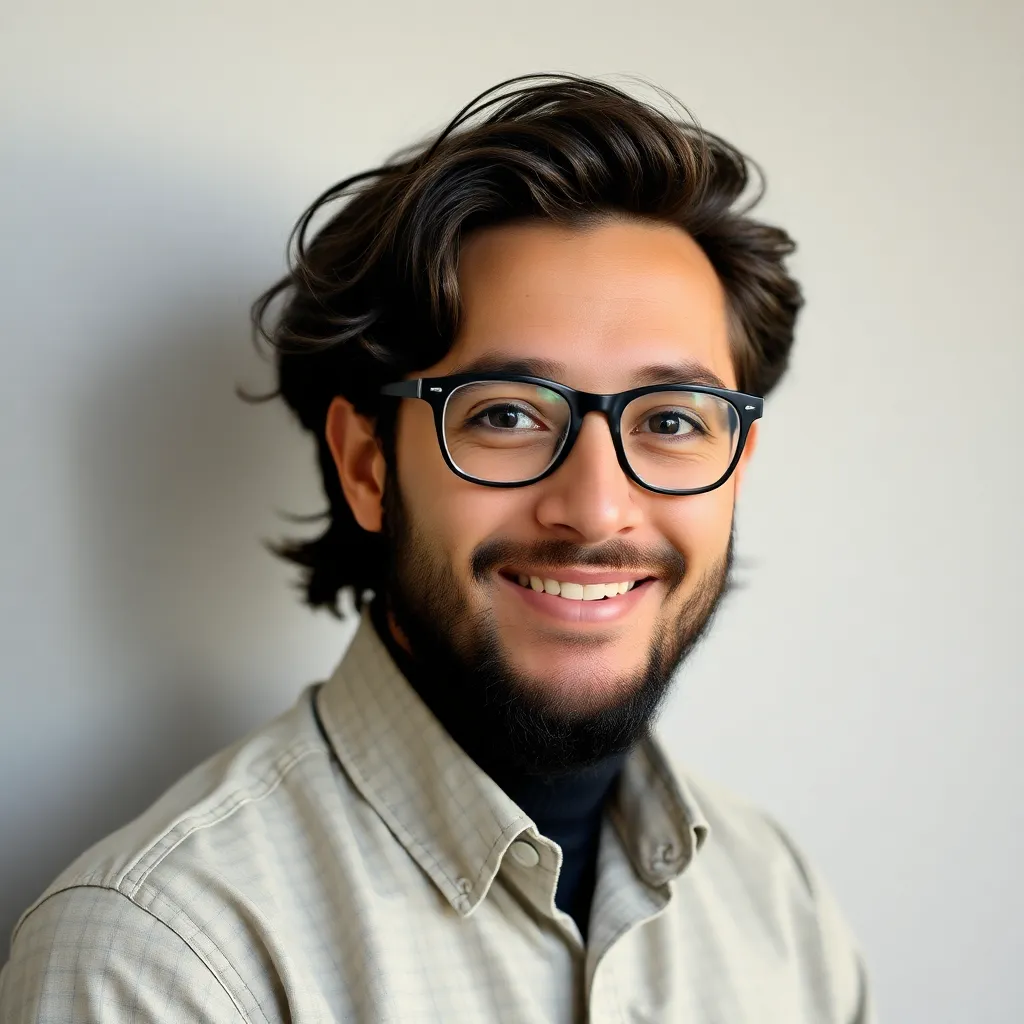
Muz Play
Apr 20, 2025 · 7 min read

Table of Contents
How Enzymes Affect Chemical Reactions: A Deep Dive into Catalysis
Enzymes are biological catalysts, vital for life as we know it. They dramatically speed up the rate of virtually all chemical reactions within cells, enabling the complex processes necessary for survival. Understanding how enzymes achieve this acceleration is crucial to grasping the fundamentals of biochemistry and its applications in medicine, biotechnology, and beyond. This article will delve deep into the mechanisms by which enzymes influence chemical reactions, exploring their structure, function, and the factors that affect their activity.
The Nature of Chemical Reactions and Activation Energy
Before diving into the role of enzymes, let's briefly review the basics of chemical reactions. Any chemical reaction involves the breaking and forming of chemical bonds. This transition from reactants to products requires overcoming an energy barrier called the activation energy (Ea). The activation energy represents the minimum energy required for the reactants to reach a transition state, an unstable intermediate state where bonds are partially broken and formed. The higher the activation energy, the slower the reaction rate because fewer molecules possess the required energy to reach the transition state.
The Role of Catalysts in Lowering Activation Energy
Catalysts, including enzymes, accelerate reaction rates without being consumed in the process. They achieve this by lowering the activation energy. Instead of the reactants needing to reach the high-energy transition state of the uncatalyzed reaction, the catalyst provides an alternative pathway with a lower activation energy. This allows more reactant molecules to overcome the energy barrier, leading to a faster reaction rate.
Enzyme Structure and Function: A Perfect Partnership
Enzymes are typically proteins, although some RNA molecules also exhibit catalytic activity (ribozymes). Their remarkable catalytic power stems from their highly specific three-dimensional structures. The unique arrangement of amino acids creates an active site, the region of the enzyme where the substrate (the molecule being acted upon) binds.
The Active Site: A Pocket of Precision
The active site is not merely a binding pocket; it's a precisely sculpted microenvironment tailored to interact with the substrate. This interaction involves several types of weak forces, including:
-
Hydrogen bonds: These weak electrostatic attractions between hydrogen atoms and electronegative atoms (like oxygen or nitrogen) play a critical role in substrate binding and orientation.
-
Hydrophobic interactions: Nonpolar regions of the substrate and enzyme interact favorably, excluding water molecules from the active site.
-
Ionic interactions: Electrostatic attractions between charged groups on the substrate and enzyme contribute to binding specificity.
-
Van der Waals forces: These weak, transient attractions arise from temporary fluctuations in electron distribution. While individually weak, their cumulative effect can be significant.
The precise arrangement of these interactions within the active site ensures high substrate specificity, meaning the enzyme catalyzes only a particular reaction with a particular substrate or a very limited range of substrates. This specificity is essential for maintaining cellular order and preventing unwanted reactions.
The Induced Fit Model: A Dynamic Embrace
The widely accepted model for enzyme-substrate interaction is the induced fit model. This model posits that the enzyme's active site is not a rigid, pre-formed structure perfectly complementary to the substrate. Instead, the substrate binding induces a conformational change in the enzyme, leading to a more complementary fit. This conformational change optimizes the interactions between the enzyme and substrate, facilitating catalysis.
Mechanisms of Enzyme Catalysis: Diverse Strategies for Success
Enzymes employ several strategies to lower the activation energy and accelerate reaction rates. These mechanisms often work in concert:
1. Proximity and Orientation: Bringing Reactants Together
Enzymes bring the substrates into close proximity and orient them in a way that favors the formation of the transition state. This drastically increases the probability of a reaction occurring by reducing the random collisions needed for reaction in an uncatalyzed reaction.
2. Strain and Distortion: Bending and Breaking Bonds
The enzyme's active site can induce strain or distortion in the substrate molecule. By forcing the substrate into a higher-energy conformation resembling the transition state, the enzyme lowers the activation energy required to reach that state.
3. Acid-Base Catalysis: Proton Transfer Power
Many enzymes employ acid-base catalysis, utilizing amino acid side chains that act as proton donors (acids) or acceptors (bases). These proton transfers can help stabilize charged transition states or facilitate the breaking or forming of bonds.
4. Covalent Catalysis: Transient Bonds for Efficiency
In covalent catalysis, a transient covalent bond forms between the enzyme and the substrate. This intermediate step provides an alternative reaction pathway with a lower activation energy than the uncatalyzed reaction. The covalent bond is subsequently broken, regenerating the enzyme and releasing the product.
5. Metal Ion Catalysis: Charge Stabilization and Redox Reactions
Metal ions, often bound to the active site, can participate in catalysis in several ways. They can stabilize charged transition states, participate in redox reactions, or act as Lewis acids, accepting electron pairs from the substrate.
Factors Affecting Enzyme Activity: A Delicate Balance
Enzyme activity is influenced by several factors, which can either enhance or inhibit their catalytic capabilities.
1. Temperature: Finding the Goldilocks Zone
Enzymes have an optimal temperature at which their activity is maximal. At lower temperatures, enzyme activity is reduced due to slower molecular motion and reduced collision frequency. At higher temperatures, excessive heat can denature the enzyme, disrupting its three-dimensional structure and destroying its catalytic activity.
2. pH: The Importance of Protonation
Enzymes also have an optimal pH range. Changes in pH can alter the charge distribution on amino acid side chains, affecting substrate binding and catalytic mechanisms. Extremes of pH can also denature the enzyme.
3. Substrate Concentration: Saturation Kinetics
As substrate concentration increases, the rate of the enzyme-catalyzed reaction increases until it reaches a maximum velocity (Vmax). At Vmax, all the enzyme active sites are saturated with substrate, and increasing substrate concentration will not further increase the reaction rate. This relationship is described by the Michaelis-Menten equation, a fundamental concept in enzyme kinetics.
4. Enzyme Concentration: More Enzymes, More Action
Increasing the concentration of the enzyme increases the reaction rate, assuming there is sufficient substrate available. More enzyme molecules mean more active sites to catalyze the reaction.
5. Inhibitors: Blocking the Action
Enzyme inhibitors are molecules that bind to the enzyme and reduce its activity. There are several types of inhibitors, including:
-
Competitive inhibitors: These inhibitors compete with the substrate for binding to the active site.
-
Noncompetitive inhibitors: These inhibitors bind to a site on the enzyme other than the active site, causing a conformational change that reduces enzyme activity.
-
Uncompetitive inhibitors: These inhibitors bind only to the enzyme-substrate complex, preventing the release of products.
The Significance of Enzymes in Biological Systems and Beyond
Enzymes are essential for virtually all biological processes. They play critical roles in:
-
Metabolism: Enzymes catalyze the countless reactions involved in energy production, nutrient breakdown, and biosynthesis.
-
DNA replication and repair: Enzymes are crucial for copying and repairing DNA, ensuring the faithful transmission of genetic information.
-
Protein synthesis: Enzymes drive the complex process of translating genetic information into proteins.
-
Signal transduction: Enzymes participate in signal transduction pathways, allowing cells to respond to their environment.
Beyond their biological roles, enzymes have numerous applications in various fields:
-
Medicine: Enzymes are used as diagnostic tools and therapeutic agents.
-
Biotechnology: Enzymes are used in industrial processes, such as the production of biofuels, pharmaceuticals, and food products.
-
Environmental science: Enzymes are used in bioremediation to clean up pollutants.
Conclusion: A Catalyst for Understanding
Enzymes are remarkable biological catalysts that dramatically speed up chemical reactions within cells. Their highly specific three-dimensional structures, coupled with diverse catalytic mechanisms, enable them to perform a myriad of essential functions. Understanding how enzymes affect chemical reactions provides invaluable insights into the intricacies of life itself and opens doors to innovative applications in diverse fields. Continued research into enzyme structure, function, and regulation promises to yield further breakthroughs in medicine, biotechnology, and beyond, further solidifying their position as pivotal players in the chemical symphony of life.
Latest Posts
Latest Posts
-
Electric Field For Infinite Line Of Charge
Apr 20, 2025
-
How To Increase Water Boiling Point
Apr 20, 2025
-
The Natural Tendency Is For Entropy To Over Time
Apr 20, 2025
-
A Carbon Atom Is Most Likely To Form
Apr 20, 2025
-
Which Statement Best Describes The Difference Between Xylem And Phloem
Apr 20, 2025
Related Post
Thank you for visiting our website which covers about How Does An Enzyme Affect A Chemical Reaction . We hope the information provided has been useful to you. Feel free to contact us if you have any questions or need further assistance. See you next time and don't miss to bookmark.