How Does Ph Affect Protein Structure
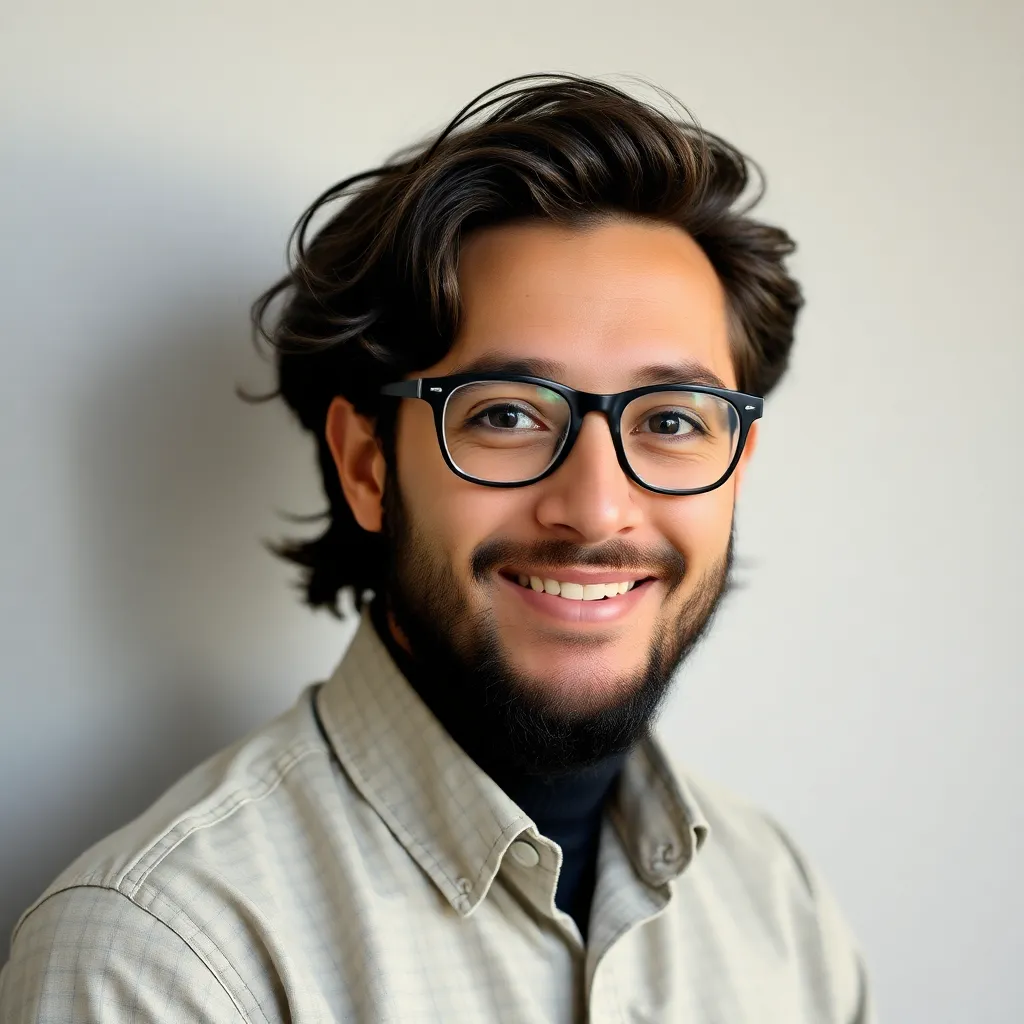
Muz Play
Apr 11, 2025 · 7 min read

Table of Contents
How Does pH Affect Protein Structure?
Proteins are the workhorses of life, carrying out a vast array of crucial functions within living organisms. Their ability to perform these diverse roles hinges critically on their three-dimensional structure, a complex arrangement dictated by the amino acid sequence and influenced significantly by environmental factors. Among these factors, pH plays a pivotal role, profoundly impacting protein structure and, consequently, function. Understanding this relationship is fundamental to comprehending numerous biological processes and developing biotechnological applications.
The Chemistry of Amino Acids and pH
Proteins are polymers composed of amino acids linked together by peptide bonds. Each amino acid possesses a carboxyl group (-COOH) and an amino group (-NH2), both capable of ionization depending on the surrounding pH. The side chain (R-group) of each amino acid also contributes to its overall charge and reactivity.
At low pH (acidic conditions), the carboxyl group (-COOH) is protonated (-COOH), while the amino group (-NH3+) remains protonated. This results in a net positive charge on the amino acid. Conversely, at high pH (alkaline conditions), the carboxyl group is deprotonated (-COO-), while the amino group loses its proton (-NH2), leading to a net negative charge.
The isoelectric point (pI) is the pH at which the net charge of an amino acid is zero. This is a crucial characteristic, as it determines the amino acid's behavior in an electric field and its interactions with other molecules. The pI varies significantly among different amino acids depending on the nature of their side chains. Some side chains possess ionizable groups, further complicating the charge distribution at different pH values.
The Impact of pH on Charge Distribution
The ionization state of amino acid side chains is profoundly affected by pH. For instance, the side chain of aspartic acid (Asp) and glutamic acid (Glu) carries a carboxyl group, which deprotonates at higher pH values, contributing to a negative charge. Conversely, the side chains of lysine (Lys), arginine (Arg), and histidine (His) possess amino groups that become protonated at lower pH values, contributing to a positive charge. Tyrosine (Tyr) and cysteine (Cys) also have ionizable side chains, further influencing the protein's overall charge distribution.
pH and Protein Structure: A Multi-Level Effect
The impact of pH on protein structure is multifaceted, influencing its various levels of organization:
1. Primary Structure: The Amino Acid Sequence
While pH does not directly alter the amino acid sequence (primary structure), it significantly influences the interactions that shape higher-order structures. Changes in charge distribution due to pH alterations affect the interactions between amino acid side chains, subsequently influencing the folding pattern and stability of the protein.
2. Secondary Structure: Alpha-Helices and Beta-Sheets
Secondary structure refers to local folding patterns within the polypeptide chain, such as alpha-helices and beta-sheets. These structures are stabilized by hydrogen bonds between the peptide backbone atoms. pH changes affect the hydrogen bonding capacity of the peptide backbone and the charge distribution of amino acid side chains, thus impacting the stability of alpha-helices and beta-sheets. Extreme pH conditions can disrupt these hydrogen bonds, leading to unfolding or denaturation.
For example, a change in pH can alter the charge of amino acid residues involved in hydrogen bond formation, weakening or disrupting these bonds. This can result in a transition from an alpha-helix to a random coil or a different secondary structure.
3. Tertiary Structure: 3D Folding and Domains
Tertiary structure describes the overall three-dimensional arrangement of a polypeptide chain, including its spatial positioning of secondary structure elements and side chain interactions. This intricate folding is stabilized by various non-covalent interactions including:
-
Hydrophobic interactions: Nonpolar amino acid side chains cluster together in the protein's core, minimizing contact with water. Changes in pH can alter the charge distribution and hydration of these side chains, thereby affecting the strength of hydrophobic interactions.
-
Hydrogen bonds: Hydrogen bonds between various polar amino acid side chains play a significant role in stabilizing the tertiary structure. pH changes can directly affect the hydrogen bonding capacity of these side chains.
-
Ionic bonds (salt bridges): Electrostatic interactions between oppositely charged amino acid side chains contribute to the stability of the tertiary structure. pH changes alter the charge of ionizable side chains, influencing the formation and strength of these ionic bonds.
-
Disulfide bonds: Covalent bonds between cysteine residues contribute to the structural integrity of some proteins. While pH does not directly break disulfide bonds, it influences the environment surrounding them, potentially affecting their stability indirectly.
Changes in pH can disrupt these interactions, leading to unfolding or denaturation of the protein, resulting in loss of function. The specific pH range over which a protein remains stable depends on its individual amino acid composition and overall structure.
4. Quaternary Structure: Multi-Subunit Assemblies
Proteins with quaternary structure consist of multiple polypeptide chains (subunits) assembled into a functional complex. pH changes can affect the interactions between these subunits, leading to dissociation or aggregation. Changes in charge distribution on the subunit surfaces due to pH shifts can disrupt the electrostatic interactions that hold them together.
Denaturation and Renaturation
The unfolding of a protein due to pH changes is termed denaturation. This process often leads to loss of biological activity. However, for some proteins, denaturation can be reversible under certain conditions, a process known as renaturation. The ability of a protein to renature depends on various factors, including the type of protein, the extent of denaturation, and the conditions used for renaturation.
Examples of pH Effects on Protein Function
Numerous examples illustrate the profound influence of pH on protein structure and function:
-
Enzymes: Many enzymes exhibit optimal activity within a narrow pH range. Deviation from this optimal pH can alter the enzyme's conformation, reducing its catalytic efficiency or completely inactivating it. For instance, pepsin, a stomach enzyme, functions optimally at a highly acidic pH (around 2), while trypsin, a pancreatic enzyme, functions optimally at a slightly alkaline pH (around 8).
-
Membrane proteins: Membrane proteins are embedded within the cell membrane and are crucial for various functions such as transport and signaling. pH changes can affect the charge distribution of amino acid residues within these proteins, influencing their interaction with the lipid bilayer and disrupting their function.
-
Structural proteins: Structural proteins like collagen, which provides structural support in connective tissues, are also sensitive to pH changes. Changes in pH can alter their conformation and reduce their mechanical strength.
-
Antibodies: The binding affinity of antibodies to their antigens can be modulated by changes in pH. This pH sensitivity can be exploited in various applications, such as immunodiagnostics and immunotherapeutics.
Technological Applications
The understanding of pH's influence on protein structure has significant implications for various biotechnological applications:
-
Protein purification: Isoelectric focusing, a technique used to separate proteins based on their pI, exploits the effect of pH on protein charge to achieve high resolution purification.
-
Protein engineering: Modifying the amino acid sequence of a protein can alter its pI and pH sensitivity, creating variants with enhanced stability or activity under specific pH conditions.
-
Drug design: Many drugs target proteins, and their efficacy can be significantly impacted by the pH of the surrounding environment. Designing drugs that are stable and functional within the relevant physiological pH range is crucial.
-
Food processing: The stability and functionality of proteins in food products are affected by pH. Controlling pH during processing is crucial to maintain the quality and texture of food items.
Conclusion
pH plays a pivotal role in shaping protein structure and function. Its influence extends across all levels of protein organization, from the local folding patterns of secondary structures to the overall 3D arrangement of tertiary and quaternary structures. Understanding the intricate relationship between pH and protein structure is critical for advancing our knowledge in biology, medicine, and biotechnology. Future research focusing on the precise mechanisms by which pH influences protein stability and function will undoubtedly yield valuable insights and applications in various fields. The continued exploration of this complex relationship promises to unravel more secrets about the intricate workings of life and inspire innovative technological solutions.
Latest Posts
Latest Posts
-
When Thermal Energy Is Removed From Particles What Action Occurs
Apr 18, 2025
-
Atomic Mass Is Equivalent To The Number Of
Apr 18, 2025
-
Which Of These Are Examples Of Inorganic Plant Nutrients
Apr 18, 2025
-
Electron Configuration And Periodic Properties Lab
Apr 18, 2025
-
Whats The Difference Between A Solute And A Solvent
Apr 18, 2025
Related Post
Thank you for visiting our website which covers about How Does Ph Affect Protein Structure . We hope the information provided has been useful to you. Feel free to contact us if you have any questions or need further assistance. See you next time and don't miss to bookmark.