How Doping Is Done In Semiconductor
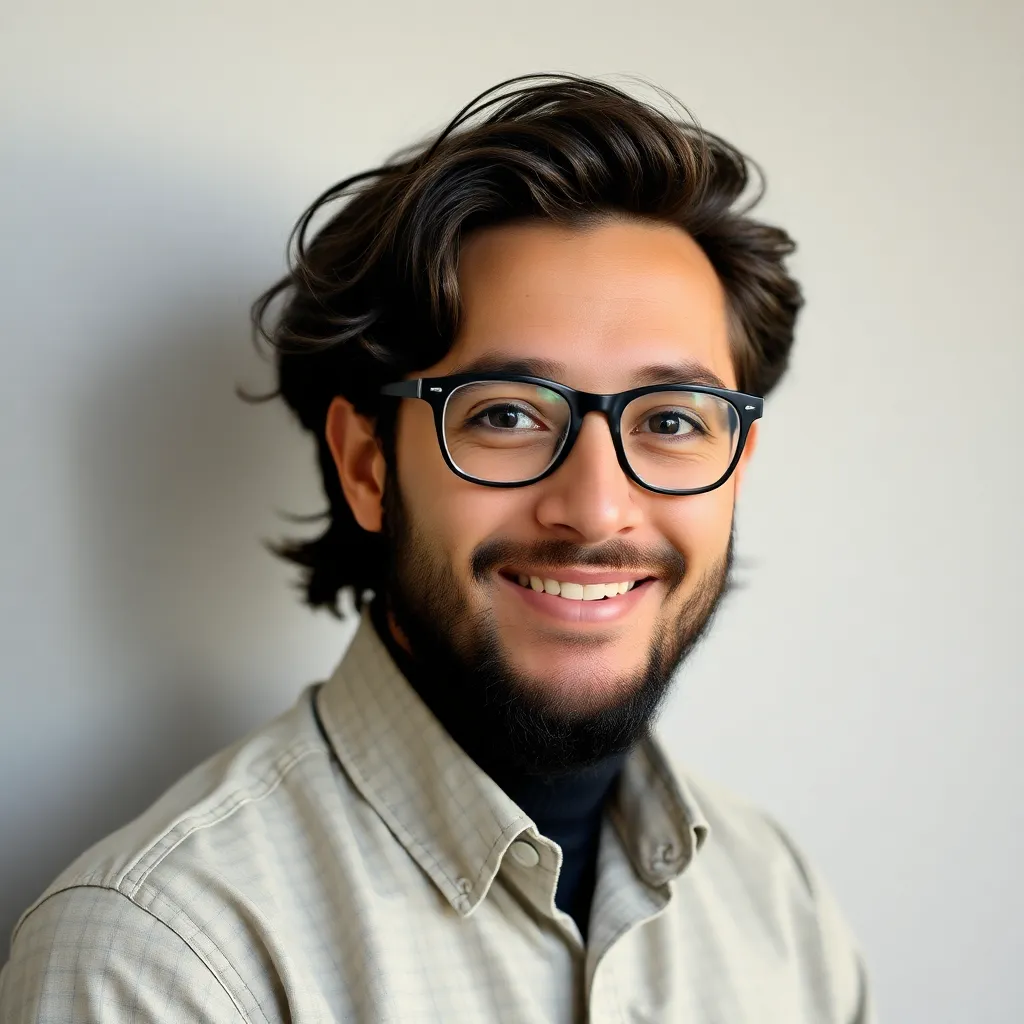
Muz Play
May 09, 2025 · 7 min read
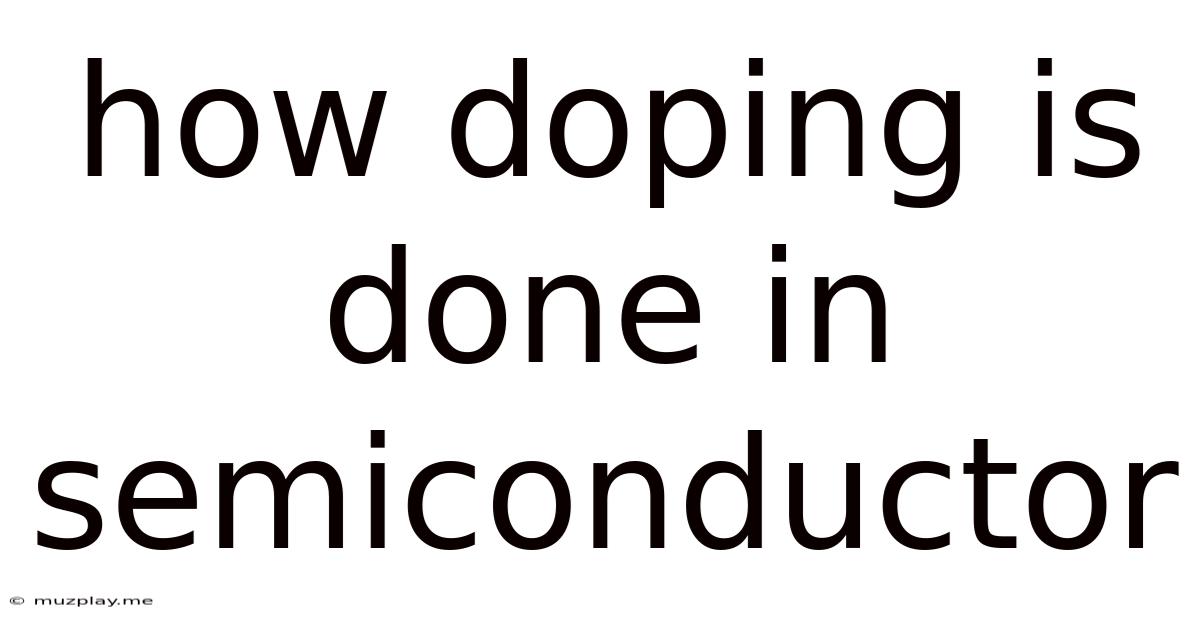
Table of Contents
How Doping Is Done in Semiconductors: A Deep Dive into Material Science
Doping is a crucial process in semiconductor manufacturing, fundamentally altering the electrical properties of a semiconductor material like silicon. By introducing controlled amounts of impurities, or dopants, we can transform a nearly-insulating material into a conductor, laying the groundwork for the transistors and integrated circuits that power our modern world. This article delves deep into the methods and mechanisms behind semiconductor doping, exploring its importance and the intricacies of the process.
Understanding the Basics: Intrinsic vs. Extrinsic Semiconductors
Before exploring doping techniques, it's essential to grasp the difference between intrinsic and extrinsic semiconductors.
Intrinsic Semiconductors: Pure and Simple
An intrinsic semiconductor, such as pure silicon (Si) or germanium (Ge), has a very low concentration of free charge carriers (electrons and holes). At absolute zero temperature, all electrons are tightly bound within their respective atoms, and the material acts as an insulator. However, at higher temperatures, some electrons gain enough thermal energy to break free from their covalent bonds, creating electron-hole pairs. These pairs contribute to a small amount of electrical conductivity. The number of free electrons equals the number of holes, maintaining electrical neutrality.
Extrinsic Semiconductors: The Power of Impurities
Extrinsic semiconductors are created by intentionally introducing impurities into the intrinsic semiconductor lattice. This process, known as doping, dramatically increases the material's conductivity and allows us to control the type and concentration of charge carriers. The type of impurity added determines the type of extrinsic semiconductor: n-type or p-type.
The Two Main Types of Extrinsic Semiconductors: N-type and P-type
N-type Semiconductors: An Excess of Electrons
N-type semiconductors are created by doping an intrinsic semiconductor with pentavalent impurities, which have five valence electrons (e.g., phosphorus (P), arsenic (As), antimony (Sb)). When a pentavalent atom replaces a silicon atom in the lattice, four of its valence electrons participate in covalent bonds with neighboring silicon atoms. The fifth electron is loosely bound and easily becomes a free electron, significantly increasing the material's electron concentration. These extra electrons are the majority carriers, while holes are the minority carriers. The pentavalent impurity atom becomes a donor because it donates an electron.
P-type Semiconductors: A Deficiency of Electrons (Excess of Holes)
P-type semiconductors are created by doping an intrinsic semiconductor with trivalent impurities, which have three valence electrons (e.g., boron (B), gallium (Ga), indium (In)). When a trivalent atom replaces a silicon atom, it forms covalent bonds with only three of its neighbors. This leaves a "hole" or vacancy in the covalent bond structure. An electron from a neighboring silicon atom can easily fill this hole, leaving behind another hole. This process effectively moves the hole through the lattice. Holes act as positive charge carriers, hence the name "p-type." The trivalent impurity atom becomes an acceptor because it accepts an electron.
Methods of Doping Semiconductors
Several techniques are employed to introduce dopants into the semiconductor lattice during manufacturing. The choice of method depends on factors like the desired doping concentration, the type of semiconductor, and the overall process requirements.
1. Ion Implantation: Precise and Controllable
Ion implantation is a highly precise technique that uses an ion accelerator to bombard the semiconductor wafer with ions of the dopant element. The energy of the ions determines their penetration depth, allowing for precise control over the doping profile. This technique is crucial for creating shallow junctions necessary for modern transistors. Following implantation, a high-temperature annealing step is needed to activate the implanted ions, which means allowing them to occupy substitutional sites in the crystal lattice and become electrically active.
2. Diffusion: A More Traditional Approach
Diffusion involves exposing the semiconductor wafer to a gaseous atmosphere containing the dopant element at high temperatures. The dopant atoms diffuse into the wafer, driven by the concentration gradient. This method is simpler than ion implantation but offers less control over the doping profile. It's often used for creating deeper junctions or for doping large areas of the wafer uniformly. The diffusion process is heavily influenced by temperature, time, and the dopant concentration in the ambient gas. The diffusion coefficient and activation energy influence the speed and depth of dopant penetration.
3. Epitaxy: Layer-by-Layer Precision
Epitaxy involves growing a thin layer of doped semiconductor material on top of an undoped substrate. This technique is used to create very precise doping profiles and allows for the integration of different semiconductor materials with controlled doping levels. Various epitaxial techniques exist, including chemical vapor deposition (CVD), molecular beam epitaxy (MBE), and liquid-phase epitaxy (LPE). These methods permit the growth of highly crystalline layers with exceptional control over the thickness and doping concentration.
4. Spin-on Doping: A Solution-Based Approach
Spin-on doping (SOD) is a relatively simple method where a solution containing the dopant is applied to the semiconductor wafer. The wafer is then spun at high speed to distribute the solution uniformly and then baked to evaporate the solvent and activate the dopant. This method is often used for localized doping or creating shallow junctions. While precise control might be less compared to ion implantation, it’s cost-effective and suitable for certain applications.
Controlling Doping Concentration and Profile
The precise control of doping concentration and profile is paramount in semiconductor device fabrication. This control is achieved through a combination of factors:
- Dopant concentration in the source material: The amount of dopant in the gas, liquid, or ion beam directly impacts the final doping level.
- Temperature and time: Diffusion and annealing processes are highly temperature and time-dependent. Higher temperatures and longer times lead to deeper penetration and higher concentrations.
- Ion implantation energy and dose: In ion implantation, the energy determines the depth of penetration, while the dose determines the dopant concentration.
- Masking techniques: Photolithography is used to create patterns on the wafer, allowing selective doping of specific regions.
Challenges and Advancements in Doping Techniques
Despite significant advances, several challenges remain in doping techniques:
- Dopant activation: Not all implanted or diffused dopant atoms become electrically active. Factors like crystal defects and clustering can reduce the activation efficiency.
- Dopant segregation: Dopants may segregate to grain boundaries or defects, leading to uneven doping profiles.
- Process control and reproducibility: Maintaining precise control over doping concentration and profile across large wafers is challenging.
- Scaling down to nanoscale dimensions: Doping at the nanoscale presents unique challenges, requiring highly precise and controlled techniques.
Significant research focuses on improving doping techniques to meet the demands of ever-shrinking semiconductor devices. This includes advancements in:
- Ultra-shallow junction formation: Creating extremely shallow junctions is crucial for high-performance transistors. Techniques like plasma doping and ultra-high vacuum ion implantation are being explored.
- Precise control of dopant profile: Advanced modeling and simulation techniques are used to optimize doping processes and achieve highly precise profiles.
- Low-temperature doping: Low-temperature processes minimize dopant diffusion and reduce damage to the semiconductor lattice.
- Novel doping materials: Researchers are investigating new dopant materials with improved properties, such as reduced diffusion and enhanced activation.
Conclusion: Doping – The Foundation of Modern Electronics
Doping is a fundamental process in semiconductor manufacturing, enabling the creation of n-type and p-type semiconductors, which are the building blocks of modern electronics. The precise control of doping concentration and profile is crucial for the performance and reliability of semiconductor devices. Ongoing research into improved doping techniques will continue to drive advancements in microelectronics, pushing the boundaries of miniaturization and performance. The techniques discussed here – ion implantation, diffusion, epitaxy, and spin-on doping – represent the core methods, with each playing a vital role depending on the specific application and required level of precision. Understanding the subtleties of doping processes is key to appreciating the incredible complexity and sophistication of modern semiconductor technology. The constant drive for improvement in these methods is what fuels the ongoing innovation in the electronics industry.
Latest Posts
Latest Posts
-
Is Xenon A Metal Nonmetal Or Metalloid
May 09, 2025
-
Which Compound Is Most Likely Formed Using Covalent Bonds
May 09, 2025
-
Difference Between Internal And External Fertilisation
May 09, 2025
-
Boron How Many Protons Neutrons And Electrons
May 09, 2025
-
Flexes The Forearm At The Elbow
May 09, 2025
Related Post
Thank you for visiting our website which covers about How Doping Is Done In Semiconductor . We hope the information provided has been useful to you. Feel free to contact us if you have any questions or need further assistance. See you next time and don't miss to bookmark.