How Is Relatedness Between Organisms And Populations Determined
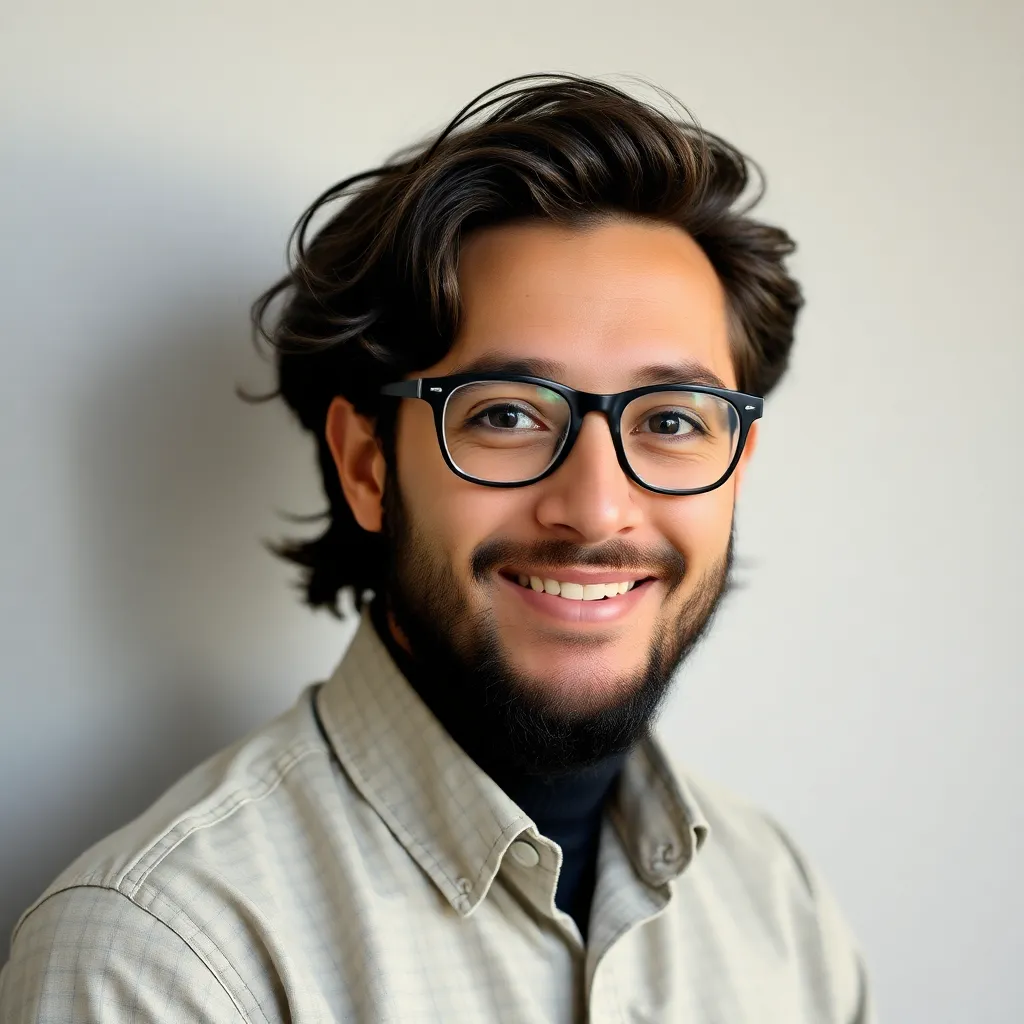
Muz Play
Mar 13, 2025 · 5 min read

Table of Contents
How is Relatedness Between Organisms and Populations Determined?
Understanding the relatedness between organisms and populations is fundamental to many areas of biology, including evolutionary biology, conservation biology, and epidemiology. Determining these relationships allows us to reconstruct evolutionary histories, understand the spread of diseases, and manage biodiversity effectively. This article explores the various methods used to determine relatedness, from traditional morphological comparisons to sophisticated molecular techniques.
Morphological Approaches: A Historical Perspective
Historically, relatedness was primarily assessed through morphological comparisons. This involved examining the physical characteristics of organisms, such as their shape, size, color, and anatomical features. The assumption was that closely related organisms would share more similar traits due to their shared ancestry. This method, while intuitively appealing, has limitations:
Limitations of Morphological Comparisons:
-
Convergent Evolution: Unrelated organisms can evolve similar traits independently in response to similar environmental pressures. This phenomenon, known as convergent evolution, can lead to misleading assessments of relatedness. For example, the streamlined bodies of dolphins (mammals) and sharks (fish) are analogous structures, not homologous structures reflecting shared ancestry.
-
Homoplasy: This refers to similarities in traits that are not due to shared ancestry but arise from other factors such as convergent evolution or parallel evolution (independent evolution of similar traits in closely related lineages). Distinguishing homoplasy from homology (similarity due to shared ancestry) is crucial for accurate phylogenetic reconstruction.
-
Plasticity: The environment can significantly influence an organism's phenotype (observable characteristics). This phenotypic plasticity can obscure underlying genetic relatedness, making morphological comparisons less reliable.
Molecular Approaches: The Rise of Genetics
The advent of molecular biology revolutionized the study of relatedness. Techniques focusing on DNA and protein sequences provide a much more precise and objective measure of relatedness compared to morphological approaches.
DNA Sequencing: The Gold Standard
DNA sequencing, the process of determining the precise order of nucleotides in a DNA molecule, is now the most widely used method for assessing relatedness. Different regions of the genome can be sequenced, each providing insights into different aspects of relatedness:
-
Mitochondrial DNA (mtDNA): MtDNA is inherited maternally and evolves relatively rapidly, making it useful for studying recent evolutionary events and population-level relationships. Its smaller size and haploid nature (only one copy per mitochondrion) simplify analysis.
-
Nuclear DNA: Nuclear DNA contains a vast amount of genetic information, and different regions evolve at different rates. This allows researchers to study relatedness across a wide range of timescales. Nuclear markers can also reveal more complex relationships than mtDNA alone.
-
Specific Genes: Certain genes evolve at predictable rates and are useful for building phylogenetic trees. Examples include ribosomal RNA genes (rRNA) and certain protein-coding genes.
Phylogenetic Analyses: Building Evolutionary Trees
The sequence data obtained from DNA sequencing are used to construct phylogenetic trees, which are visual representations of the evolutionary relationships between organisms or populations. Several computational methods are used to build these trees, including:
-
Maximum Parsimony: This method seeks the tree that requires the fewest evolutionary changes to explain the observed data.
-
Maximum Likelihood: This method calculates the probability of observing the data given a particular tree, selecting the tree with the highest probability.
-
Bayesian Inference: This method uses Bayesian statistics to infer the posterior probability of different trees, incorporating prior knowledge about evolutionary rates and processes.
Other Molecular Markers:
Beyond DNA sequencing, other molecular markers provide insights into relatedness:
-
Microsatellites (Short Tandem Repeats): These are short, repetitive DNA sequences that vary in length among individuals. They are highly polymorphic (have many different alleles) and useful for studying fine-scale population structure and relatedness within populations.
-
Single Nucleotide Polymorphisms (SNPs): These are single base-pair changes in DNA sequence. SNPs are abundant in genomes and can be used to study relatedness at both population and individual levels.
-
Restriction Fragment Length Polymorphisms (RFLPs): This technique involves cutting DNA with restriction enzymes and analyzing the resulting fragments. While less commonly used now compared to direct sequencing, it remains a valuable tool in certain contexts.
-
Protein Electrophoresis: This technique separates proteins based on their size and charge, revealing variations between individuals or populations. While less informative than DNA sequencing, it can be used as a complementary approach.
Combining Approaches: A Holistic View
The most powerful approach to determining relatedness often involves combining morphological and molecular data. Molecular data provide a quantitative and objective measure of relatedness, while morphological data can provide context and insights into the functional significance of observed genetic differences. This integrated approach helps to overcome the limitations of each individual method.
Applications of Relatedness Studies:
Understanding relatedness has crucial implications across various biological disciplines:
-
Conservation Biology: Determining the genetic diversity and relatedness of populations is essential for developing effective conservation strategies. Identifying genetically distinct populations allows for targeted conservation efforts and the avoidance of inbreeding.
-
Epidemiology: Tracing the spread of infectious diseases requires understanding the relatedness of different strains of pathogens. Phylogenetic analyses can reveal the origin of outbreaks and help in predicting future outbreaks.
-
Forensic Science: DNA profiling is used to determine relatedness between individuals, which has applications in paternity testing and criminal investigations.
-
Evolutionary Biology: Understanding relatedness is fundamental to reconstructing the evolutionary history of life on Earth. Phylogenetic analyses allow us to understand the relationships between different species and trace their evolutionary pathways.
-
Anthropology and Archaeology: Studying the relatedness of ancient human populations helps to understand human migrations and population history.
Challenges and Future Directions:
Despite the significant advances in molecular techniques, several challenges remain:
-
Incomplete Lineage Sorting: The inheritance of genetic material is not always consistent across generations, leading to incomplete lineage sorting, where different genes show conflicting phylogenetic signals.
-
Horizontal Gene Transfer: In some organisms, particularly prokaryotes, genes can be transferred horizontally between unrelated individuals. This can complicate the interpretation of phylogenetic trees.
-
Data Analysis and Interpretation: Analyzing large molecular datasets requires sophisticated computational tools and expertise. Interpreting the results requires a strong understanding of evolutionary processes.
The future of relatedness studies involves integrating even larger datasets, incorporating more sophisticated analytical methods, and combining genomic data with other sources of information, such as ecological data and phenotypic information. The development of new technologies, such as high-throughput sequencing and advanced bioinformatics tools, will continue to drive progress in this field, allowing for increasingly precise and comprehensive assessments of relatedness between organisms and populations. This improved understanding will have profound implications for our ability to address critical challenges in biodiversity conservation, disease control, and our understanding of the history of life itself.
Latest Posts
Latest Posts
-
What Is The Magnification Power Of The Low Objective Lens
May 09, 2025
-
Atom With Atomic Number Equal To 2
May 09, 2025
-
Como Se Forma Las Rocas Igneas
May 09, 2025
-
Where Does Fertilization Take Place In The Flower
May 09, 2025
-
Is Argon A Metal Or Nonmetal
May 09, 2025
Related Post
Thank you for visiting our website which covers about How Is Relatedness Between Organisms And Populations Determined . We hope the information provided has been useful to you. Feel free to contact us if you have any questions or need further assistance. See you next time and don't miss to bookmark.