How Many Electrons Can 3d Hold
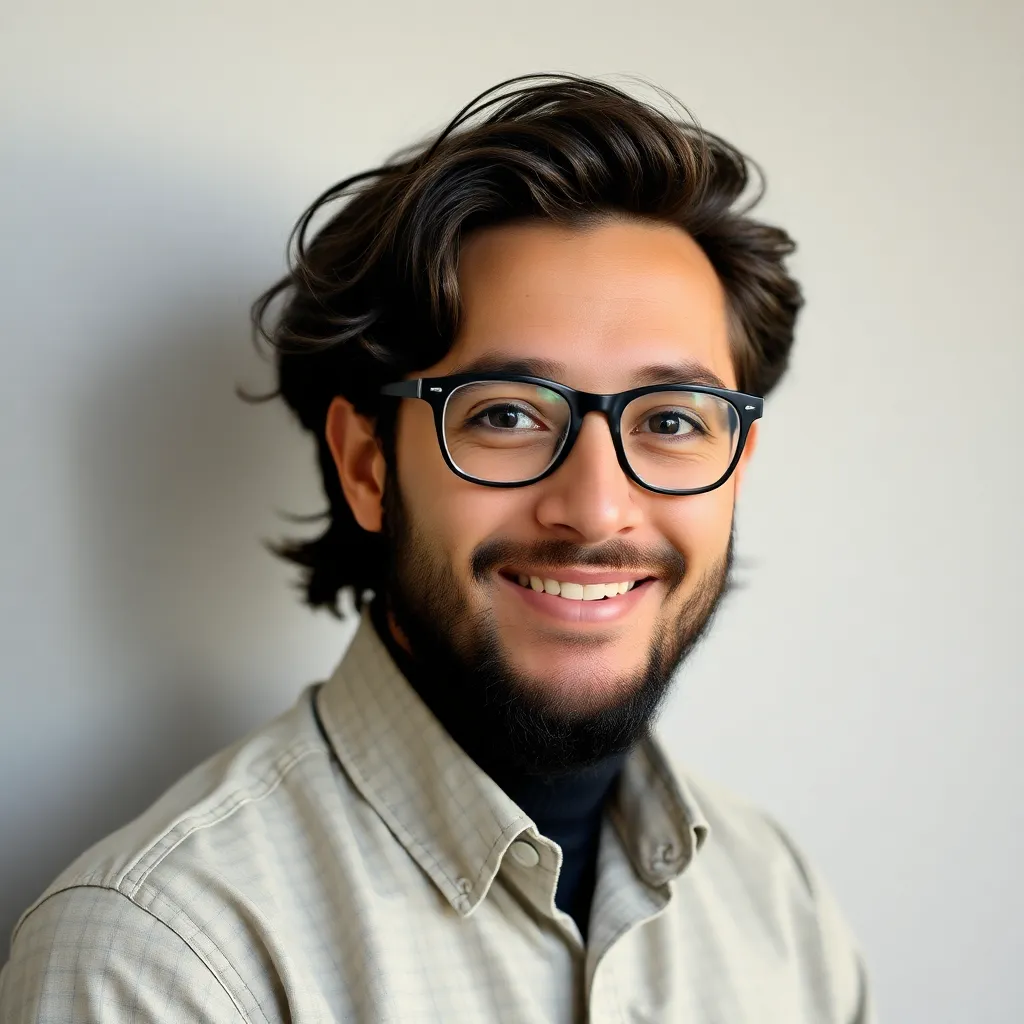
Muz Play
May 09, 2025 · 6 min read
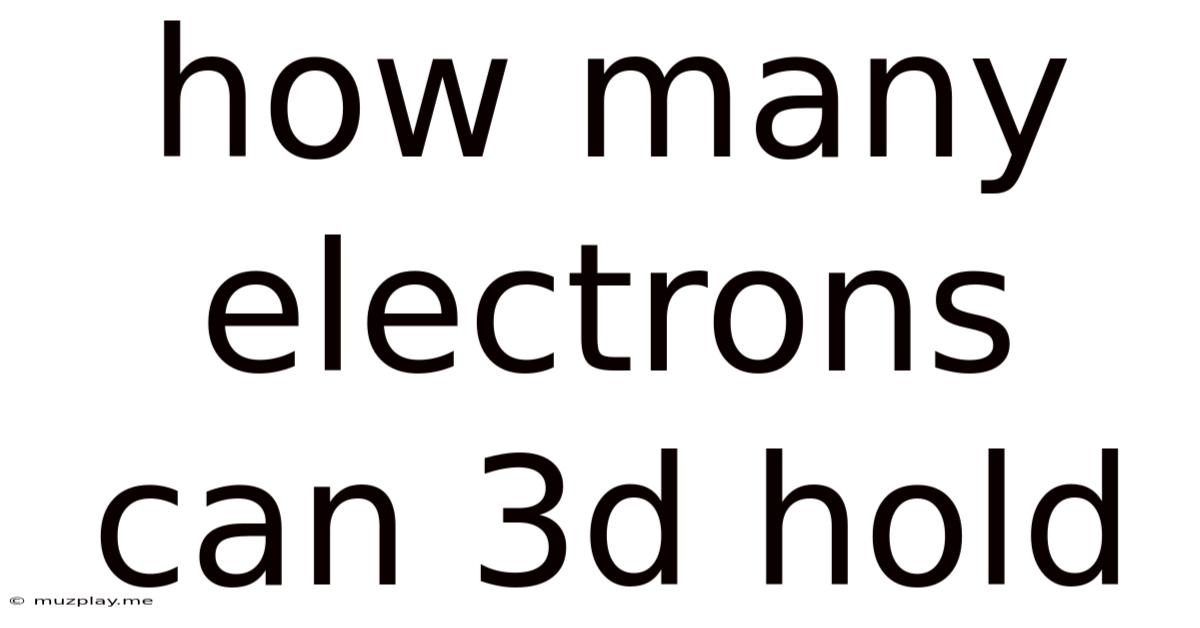
Table of Contents
How Many Electrons Can a 3d Orbital Hold? A Deep Dive into Electron Configuration
Understanding electron configuration is fundamental to comprehending the behavior of atoms and molecules. A key aspect of this is knowing the electron capacity of different orbitals. This article will delve deep into the question: how many electrons can a 3d orbital hold? We'll explore the underlying principles of quantum mechanics, the structure of the 3d subshell, and the implications of its electron capacity on the properties of elements.
Understanding Electron Shells, Subshells, and Orbitals
Before tackling the 3d orbital specifically, let's establish a foundational understanding of atomic structure. Electrons don't simply orbit the nucleus randomly; they exist in specific energy levels, organized into shells, subshells, and orbitals.
-
Electron Shells (Principal Quantum Number, n): These represent the main energy levels of an electron. They are numbered sequentially (n = 1, 2, 3, etc.), with higher numbers indicating greater energy and distance from the nucleus. The shell number determines the maximum number of electrons the shell can hold, using the formula 2n². For example, the first shell (n=1) can hold a maximum of 2 electrons (2 x 1² = 2).
-
Electron Subshells (Azimuthal Quantum Number, l): Within each shell, electrons are further organized into subshells, which are denoted by letters: s, p, d, and f. The number of subshells in a given shell is equal to the shell number (n). Each subshell has a specific shape and can hold a different maximum number of electrons.
- s subshell (l=0): Spherical shape. Holds a maximum of 2 electrons.
- p subshell (l=1): Dumbbell shape. Holds a maximum of 6 electrons.
- d subshell (l=2): More complex shape. Holds a maximum of 10 electrons.
- f subshell (l=3): Even more complex shape. Holds a maximum of 14 electrons.
-
Electron Orbitals (Magnetic Quantum Number, ml): Each subshell contains one or more orbitals. Orbitals are regions of space where there's a high probability of finding an electron. The number of orbitals in each subshell is determined by the subshell letter:
- s subshell: 1 orbital
- p subshell: 3 orbitals
- d subshell: 5 orbitals
- f subshell: 7 orbitals
The 3d Subshell: Structure and Electron Capacity
Now, let's focus on the 3d subshell. This subshell is part of the third electron shell (n=3) and contains five 3d orbitals. Each orbital can hold a maximum of two electrons according to the Pauli Exclusion Principle, which states that no two electrons in an atom can have the same set of four quantum numbers. This principle incorporates the spin quantum number (ms), which can have two values: +1/2 (spin up) and -1/2 (spin down).
Therefore, since the 3d subshell has five orbitals, and each orbital can hold two electrons, the maximum number of electrons that the 3d subshell can hold is 10.
Filling the 3d Subshell: Hund's Rule and Electron Configuration
The order in which electrons fill the orbitals within the 3d subshell follows specific rules:
-
Aufbau Principle: Electrons fill orbitals starting from the lowest energy level and moving upwards. Generally, the 4s subshell fills before the 3d subshell, but there are exceptions.
-
Hund's Rule: Electrons will individually occupy each orbital within a subshell before doubling up in any one orbital. This minimizes electron-electron repulsion. Each orbital will first receive one electron with the same spin before pairing up with an electron of opposite spin.
Example: Iron (Fe)
Iron has an atomic number of 26, meaning it has 26 electrons. Its electron configuration is 1s²2s²2p⁶3s²3p⁶4s²3d⁶. Notice that the 4s subshell fills before the 3d subshell. The 3d subshell contains 6 electrons, distributed according to Hund's Rule, with four orbitals singly occupied and one orbital doubly occupied.
The Significance of the 3d Electron Capacity
The capacity of the 3d subshell to hold ten electrons has profound implications for the properties of elements, particularly those in the transition metal series. These elements are characterized by partially filled 3d subshells, leading to several key features:
-
Variable Oxidation States: Transition metals exhibit a range of oxidation states because they can lose electrons from both the 4s and 3d subshells. This leads to diverse chemical behaviors and the formation of numerous compounds.
-
Complex Ion Formation: The partially filled 3d orbitals readily participate in coordinate covalent bonding, enabling the formation of complex ions with ligands. This property is crucial in many biological processes and industrial applications (e.g., catalysis).
-
Colored Compounds: The d-d electronic transitions within partially filled 3d orbitals result in the absorption of specific wavelengths of light, leading to the characteristic colors observed in many transition metal compounds.
-
Magnetic Properties: The presence of unpaired electrons in the 3d subshell leads to paramagnetism, where the substance is weakly attracted to a magnetic field. Some transition metal compounds exhibit ferromagnetism or antiferromagnetism, depending on the arrangement of electron spins.
-
Catalytic Activity: Transition metals, with their variable oxidation states and partially filled d-orbitals, often act as catalysts, speeding up chemical reactions without being consumed themselves. This is vital in various industrial processes and biological systems.
Exceptions to the Filling Order and Electron Configuration Anomalies
While the Aufbau principle and Hund's Rule generally predict electron configurations accurately, there are exceptions, especially involving the filling order of the 3d and 4s subshells. These exceptions arise due to subtle differences in electron-electron interactions and orbital energies. For example, chromium (Cr) and copper (Cu) have anomalous electron configurations:
-
Chromium (Cr): Instead of [Ar] 3d⁴4s², it has [Ar] 3d⁵4s¹. This half-filled 3d subshell is exceptionally stable due to electron-electron interactions.
-
Copper (Cu): Instead of [Ar] 3d⁹4s², it has [Ar] 3d¹⁰4s¹. A completely filled 3d subshell is also particularly stable.
These anomalies highlight the complexities of electron configurations and the limitations of simple rules. More sophisticated calculations considering electron-electron interactions are often required to accurately predict the ground state electron configuration for some atoms.
Conclusion: The Crucial Role of the 3d Subshell
The 3d subshell, with its capacity to hold ten electrons, plays a crucial role in determining the properties of elements, especially transition metals. Understanding its structure, filling order, and electron capacity is essential for comprehending a wide range of chemical phenomena. The exceptions to the standard filling rules further emphasize the need for deeper understanding of atomic structure and the quantum mechanical principles governing electron behavior. This knowledge provides a solid foundation for exploring more advanced topics in chemistry and physics. The behavior of electrons within the 3d orbital significantly affects the chemical and physical characteristics that define the unique identities of countless elements. The ability to predict and understand these properties allows us to better utilize these elements in various applications.
Latest Posts
Latest Posts
-
What Types Of Intermolecular Forces Are Found In H2o
May 10, 2025
-
In Eukaryotes Cellular Respiration Takes Place In The
May 10, 2025
-
How To Calculate Transmittance From Absorbance
May 10, 2025
-
During Osmosis Water Moves Blank Its Concentration Gradient
May 10, 2025
-
Mass Media As An Agent Of Socialisation
May 10, 2025
Related Post
Thank you for visiting our website which covers about How Many Electrons Can 3d Hold . We hope the information provided has been useful to you. Feel free to contact us if you have any questions or need further assistance. See you next time and don't miss to bookmark.