How Many Lines Are In The Absorption Line Spectrum
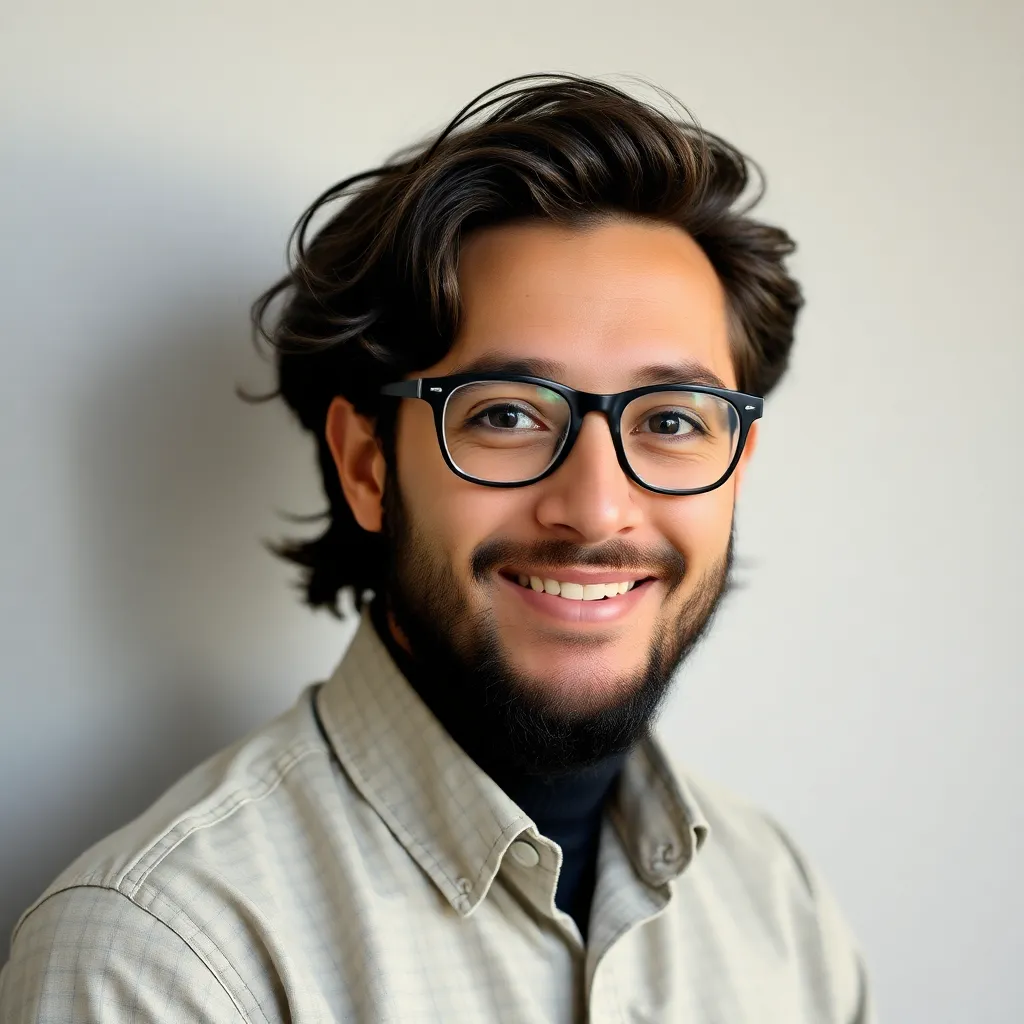
Muz Play
May 11, 2025 · 5 min read
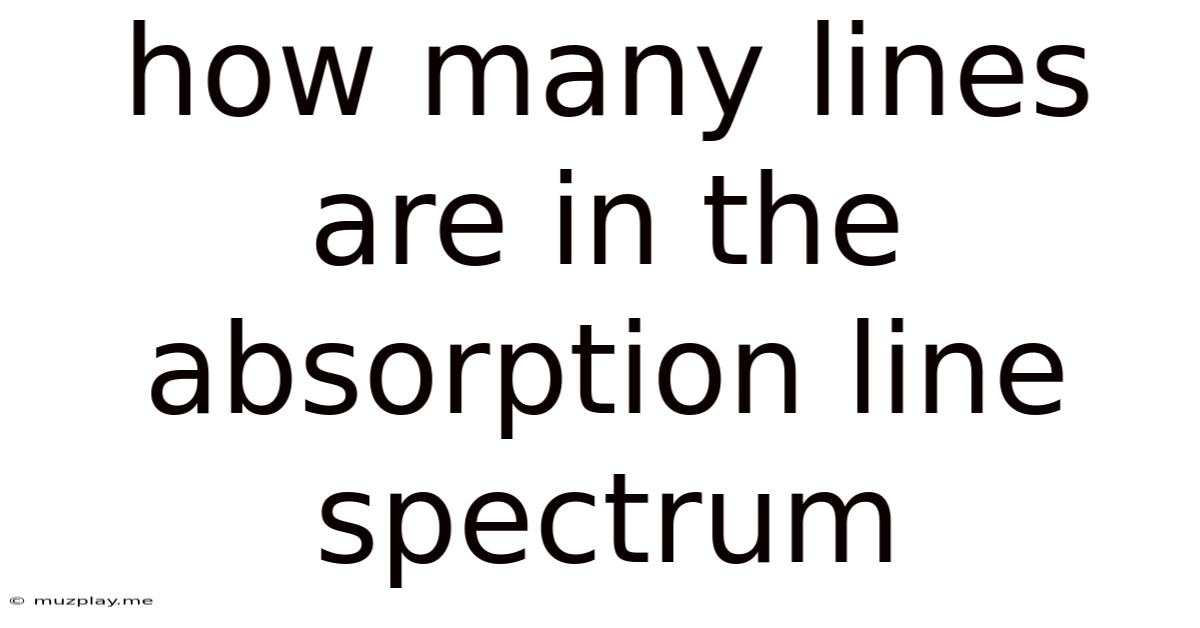
Table of Contents
How Many Lines Are in an Absorption Line Spectrum? A Deep Dive into Atomic Fingerprints
The seemingly simple question, "How many lines are in an absorption line spectrum?" unveils a surprisingly complex answer. It's not a fixed number, but rather a characteristic unique to each element and significantly influenced by factors like temperature, pressure, and the instrument's resolution. Understanding this requires delving into the fundamentals of atomic structure, spectroscopy, and the intricacies of spectral line formation.
Understanding Absorption Line Spectra
An absorption line spectrum arises when light from a continuous source (like a hot, dense body) passes through a cooler, less dense gas. Atoms in this gas absorb specific wavelengths of light, corresponding to transitions between their energy levels. These absorbed wavelengths manifest as dark lines against the continuous spectrum, hence the term "absorption lines." The number and positions of these lines act as a unique "fingerprint" for each element, a cornerstone of spectroscopy.
The Role of Electron Energy Levels
The key to understanding the number of lines lies in the quantized nature of electron energy levels within an atom. Electrons can only occupy specific energy states; they cannot exist in between. When an electron absorbs a photon of light with energy precisely matching the difference between two energy levels, it jumps to a higher energy level (excited state). This leaves a dark line in the spectrum at that specific wavelength.
Transitions and Spectral Lines
Each absorption line corresponds to a specific electron transition. The number of possible transitions, and thus the number of absorption lines, depends on several factors:
- Number of energy levels: Atoms with more electrons have more complex energy level structures, leading to a greater number of possible transitions and thus more absorption lines.
- Selection rules: Not all transitions are allowed. Quantum mechanical selection rules govern which transitions are permitted and which are forbidden. These rules restrict the changes in the quantum numbers associated with the electron's state (e.g., principal quantum number, orbital angular momentum quantum number, spin quantum number). Forbidden transitions can still occur, but with much lower probability.
- Degeneracy of energy levels: Energy levels can be degenerate, meaning they have the same energy despite differing in other quantum numbers. These degeneracies can lift under the influence of external fields (e.g., Zeeman effect due to magnetic fields, Stark effect due to electric fields), leading to further splitting of spectral lines and increasing the observed number of lines.
Factors Influencing the Number of Observed Lines
Even for a single element, the number of observed absorption lines is not fixed and can vary considerably depending on several factors:
1. Temperature and Pressure
At higher temperatures, more electrons are excited to higher energy levels, resulting in a greater number of possible transitions and therefore more absorption lines. Conversely, at lower temperatures, fewer electrons are excited, leading to a simpler spectrum with fewer lines.
Pressure also plays a role. High pressure can broaden the spectral lines, causing them to overlap and potentially obscuring some lines. This effectively reduces the number of distinctly observable lines, even though the underlying number of possible transitions remains the same.
2. Resolution of the Spectrometer
The resolving power of the spectrometer employed significantly impacts the number of lines detected. High-resolution spectrometers can distinguish between closely spaced lines that might appear as a single, broadened line in a low-resolution instrument. Improved resolution reveals finer details in the spectrum, increasing the observed number of lines.
3. Path Length
The length of the path the light travels through the absorbing gas also affects the observed spectrum. A longer path length increases the probability of absorption, resulting in stronger absorption lines and potentially making weaker lines more readily detectable.
4. Isotopes
Isotopes of the same element have the same number of protons but different numbers of neutrons. This slight difference in mass can lead to subtle shifts in energy levels and therefore slightly different positions of spectral lines. High-resolution spectroscopy can resolve these isotopic shifts, leading to an increase in the apparent number of lines observed for an element.
5. Molecular Absorption
If the absorbing gas consists of molecules instead of solely individual atoms, the situation becomes considerably more complex. Molecules possess rotational and vibrational energy levels in addition to electronic energy levels, leading to a vast increase in the number of possible transitions and therefore absorption lines. This generates a much richer and more complex spectrum. Molecular spectra often exhibit numerous closely spaced lines, making the counting of individual lines extremely challenging.
Practical Examples and Limitations
Let's consider a few examples to illustrate the variability:
-
Hydrogen: The simplest atom, hydrogen, exhibits a relatively simple spectrum with a series of lines (Lyman, Balmer, Paschen, etc.) corresponding to transitions between different energy levels. However, even for hydrogen, high-resolution spectroscopy reveals fine structure splitting due to spin-orbit coupling, significantly increasing the observed number of lines.
-
Complex Atoms: Heavier atoms with many electrons have exceedingly complex spectra with hundreds or even thousands of observable absorption lines, particularly under high resolution and elevated temperatures. It's often impractical to count every single line.
-
Molecular Spectra: The absorption spectra of molecules are far more intricate. The number of absorption lines can easily run into the tens of thousands or even more, depending on the molecule's complexity and the spectral region examined. Detailed assignments of all lines are typically only possible with advanced computational techniques.
Conclusion: Beyond Simple Counting
The question of "how many lines" doesn't have a straightforward numerical answer. The number of absorption lines observed in a spectrum depends intricately on the element or molecule, the experimental conditions (temperature, pressure, path length), the resolution of the measuring instrument, and the presence of isotopes. While simplified models can predict the main spectral lines, the true complexity is often far greater, particularly for heavier atoms and molecules. Instead of simply counting lines, spectroscopists focus on analyzing the pattern of lines, their relative intensities, and their precise wavelengths to extract meaningful information about the composition and conditions of the sample being studied. This detailed spectral analysis is crucial in diverse fields ranging from astronomy to materials science and analytical chemistry.
Latest Posts
Latest Posts
-
Volume Of Hexagonal Close Packed Unit Cell
May 12, 2025
-
According To The Life Span Perspective Human Development
May 12, 2025
-
In One Of The Reactions In The Electron Transport Chain
May 12, 2025
-
Write The Exponential Expression Using Radicals
May 12, 2025
-
Place Each Joint Characteristic Into The Classification Of Joint Type
May 12, 2025
Related Post
Thank you for visiting our website which covers about How Many Lines Are In The Absorption Line Spectrum . We hope the information provided has been useful to you. Feel free to contact us if you have any questions or need further assistance. See you next time and don't miss to bookmark.