How Much Atp Is Produced In The Electron Transport Chain
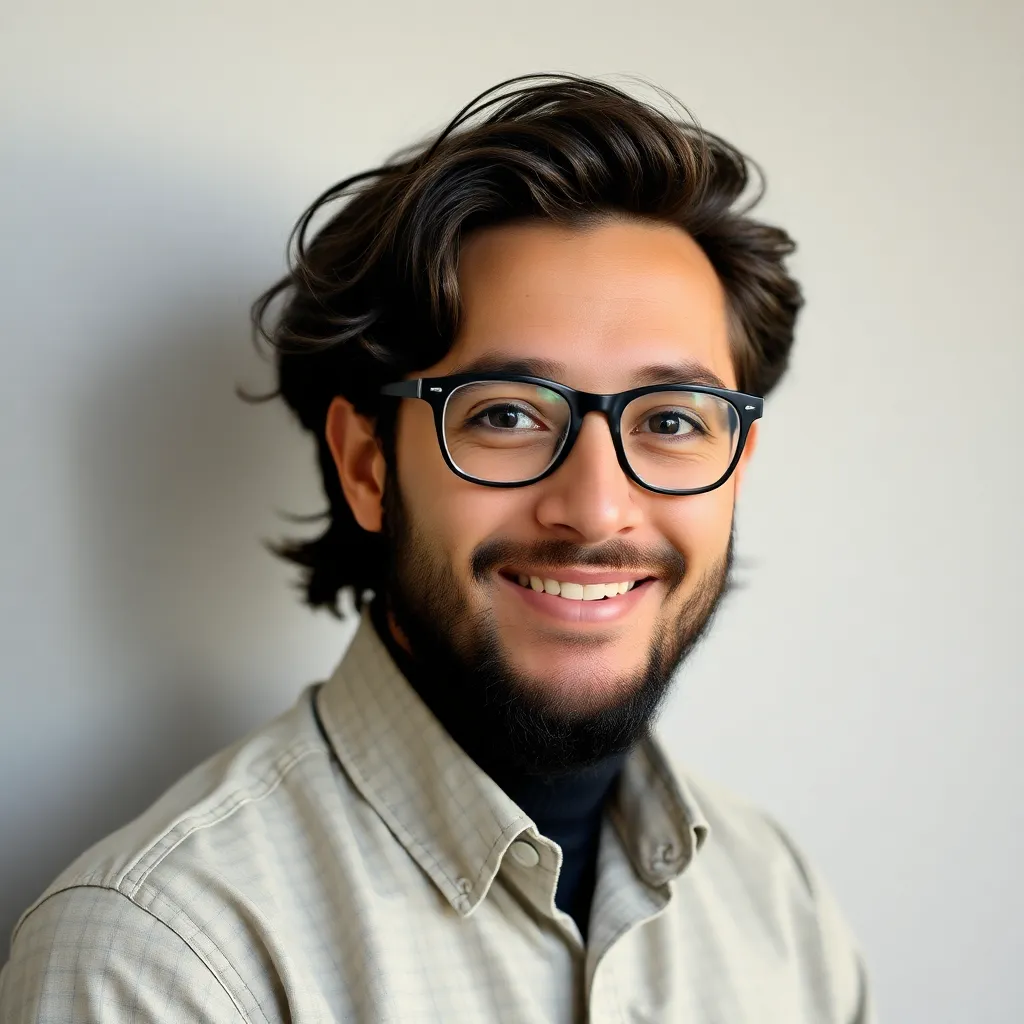
Muz Play
May 11, 2025 · 6 min read
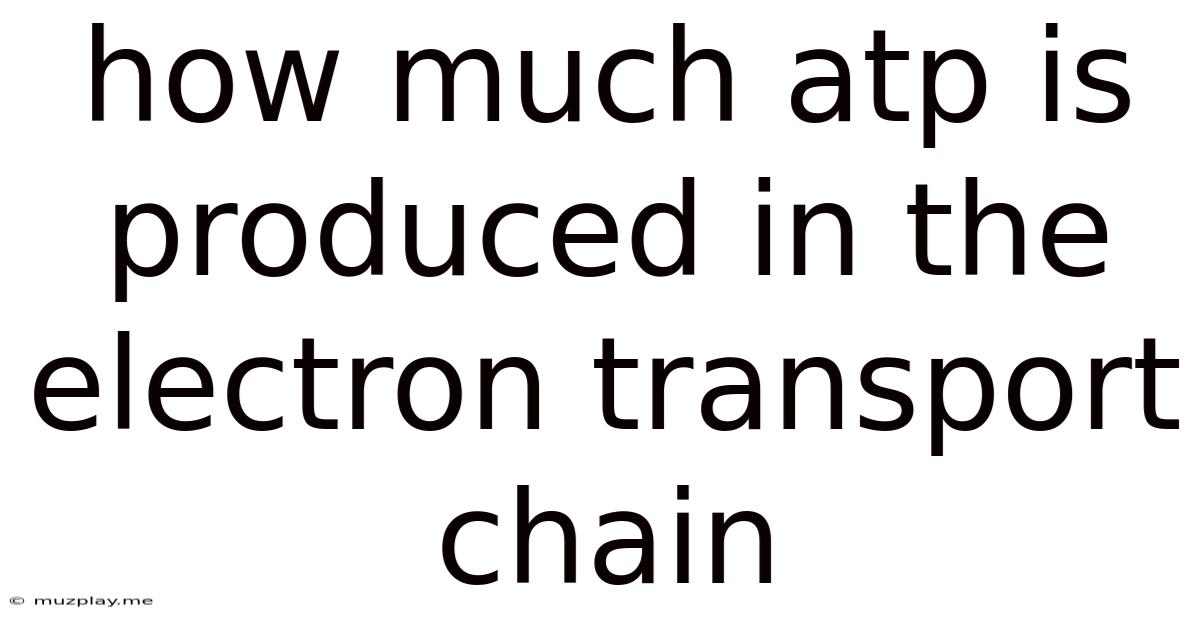
Table of Contents
How Much ATP is Produced in the Electron Transport Chain? A Deep Dive into Oxidative Phosphorylation
The electron transport chain (ETC), also known as the respiratory chain, is the final stage of cellular respiration. It's a crucial process where the energy stored in reduced electron carriers, NADH and FADH2, is used to generate a significant amount of ATP, the cell's primary energy currency. Understanding exactly how much ATP is produced requires a nuanced look at the intricate mechanisms involved. This article will delve into the complexities of oxidative phosphorylation, exploring the factors that influence ATP yield and clarifying some common misconceptions.
The Electron Transport Chain: A Cascade of Redox Reactions
The ETC is located in the inner mitochondrial membrane in eukaryotes and the plasma membrane in prokaryotes. It's a series of protein complexes (Complexes I-IV) and mobile electron carriers (ubiquinone and cytochrome c) that facilitate a controlled flow of electrons. This flow is coupled to the pumping of protons (H+) from the mitochondrial matrix to the intermembrane space, creating a proton gradient. This gradient is the driving force behind ATP synthesis.
Complex I: NADH Dehydrogenase
Complex I, also known as NADH dehydrogenase, receives electrons from NADH. These electrons are passed along a series of electron carriers within the complex, ultimately reducing ubiquinone (Q) to ubiquinol (QH2). Importantly, this electron transfer is coupled to the pumping of four protons across the membrane.
Complex II: Succinate Dehydrogenase
Complex II, succinate dehydrogenase, is slightly different. It's part of both the citric acid cycle and the ETC. It receives electrons from FADH2, a byproduct of the citric acid cycle. Unlike Complex I, Complex II does not pump protons. The electrons from FADH2 are passed to ubiquinone, bypassing the proton-pumping stage associated with Complex I.
Ubiquinone (Coenzyme Q)
Ubiquinone, a lipid-soluble molecule, acts as a mobile electron carrier, shuttling electrons from Complexes I and II to Complex III.
Complex III: Cytochrome bc1 Complex
Complex III, the cytochrome bc1 complex, receives electrons from ubiquinol (QH2). Through a process called the Q cycle, electrons are transferred to cytochrome c, another mobile electron carrier. This process also pumps protons across the membrane. The precise number of protons pumped per electron pair is debated, but it's generally considered to be four protons.
Cytochrome c
Cytochrome c, a small protein, carries electrons from Complex III to Complex IV.
Complex IV: Cytochrome c Oxidase
Complex IV, cytochrome c oxidase, is the terminal electron acceptor complex. It receives electrons from cytochrome c and ultimately transfers them to oxygen (O2), reducing it to water (H2O). This final step also pumps protons across the membrane. The number of protons pumped is generally accepted to be two protons per electron pair.
ATP Synthase: The Powerhouse of Oxidative Phosphorylation
The proton gradient generated by the ETC creates a proton-motive force (PMF). This PMF drives ATP synthesis through ATP synthase, a remarkable molecular machine embedded in the inner mitochondrial membrane. ATP synthase utilizes the energy stored in the PMF to phosphorylate ADP, converting it to ATP.
Calculating ATP Yield: A Complex Equation
Calculating the precise ATP yield from the ETC is complicated by several factors:
-
Proton stoichiometry: The exact number of protons pumped per electron pair at each complex isn't universally agreed upon. Slight variations reported in different studies can influence the overall ATP yield calculation.
-
Proton slip: Some protons may leak back across the membrane without contributing to ATP synthesis, reducing the effective PMF.
-
P/O ratio: The P/O ratio (phosphate/oxygen ratio) represents the number of ATP molecules synthesized per atom of oxygen reduced. Experimental determination of this ratio has provided valuable insights, but it's not a fixed value.
-
NADH vs. FADH2: NADH delivers electrons to Complex I, resulting in more protons pumped and therefore more ATP produced compared to FADH2, which enters the ETC at Complex II.
A Simplified Calculation:
Despite the complexities, a simplified calculation often used provides a reasonable estimate. Assuming:
- Complex I: 4 protons pumped per NADH
- Complex III: 4 protons pumped per NADH (from Q cycle)
- Complex IV: 2 protons pumped per NADH
This gives a total of 10 protons pumped per NADH. If we assume a ratio of approximately 3 protons required per ATP synthesized (this ratio varies slightly depending on the experimental approach and context), then each NADH molecule would theoretically yield approximately 3.33 ATP molecules. Since this is not a whole number, it's often rounded to 3 ATP per NADH.
For FADH2, the calculation is different, as it skips Complex I:
- Complex III: 4 protons pumped per FADH2 (from Q cycle)
- Complex IV: 2 protons pumped per FADH2
This yields a total of 6 protons pumped per FADH2. Using the same approximate 3 protons per ATP, FADH2 would produce approximately 2 ATP molecules. Again, this is often simplified to 2 ATP per FADH2.
The Role of the Citric Acid Cycle and Glycolysis
The ATP yield from the ETC is dependent on the number of NADH and FADH2 molecules produced during the preceding metabolic pathways: glycolysis and the citric acid cycle.
- Glycolysis: Produces 2 NADH per glucose molecule.
- Citric Acid Cycle: Produces 6 NADH and 2 FADH2 per glucose molecule.
Therefore, the total number of ATP generated from the ETC is the sum of ATP generated from NADH and FADH2 originating from these metabolic pathways.
Putting it All Together: Total ATP Production per Glucose Molecule
A commonly cited estimate for total ATP production per glucose molecule during cellular respiration combines ATP from glycolysis, the citric acid cycle and the electron transport chain:
- Glycolysis: 2 ATP (substrate-level phosphorylation) + 2 NADH (x 3 ATP/NADH = 6 ATP) = 8 ATP
- Citric Acid Cycle: 2 ATP (substrate-level phosphorylation) + 6 NADH (x 3 ATP/NADH = 18 ATP) + 2 FADH2 (x 2 ATP/FADH2 = 4 ATP) = 24 ATP
- Electron Transport Chain: (Referencing the NADH and FADH2 from Glycolysis and Citric Acid Cycle above)
This yields a total of approximately 32 or 34 ATP molecules per glucose molecule. The variation depends on the shuttle system used to transport cytoplasmic NADH into the mitochondria (malate-aspartate shuttle versus glycerol-3-phosphate shuttle). The malate-aspartate shuttle yields a higher ATP count.
Conclusion: A Dynamic and Complex Process
The exact number of ATP molecules produced during oxidative phosphorylation is not a static value. It's influenced by several factors, making a precise calculation challenging. The simplified calculations presented provide a reasonable approximation, highlighting the substantial contribution of the ETC to cellular energy production. Understanding the complexities of proton stoichiometry, proton slip, and shuttle systems is crucial for appreciating the intricacies of this fundamental biological process. Further research continues to refine our understanding of the precise energy yield of the electron transport chain.
Latest Posts
Latest Posts
-
How Was The First Periodic Table Arranged
May 12, 2025
-
The Laboratory Work Surface Should Be Wiped With Disinfectant
May 12, 2025
-
What Is The Difference Between Polar Protic And Polar Aprotic
May 12, 2025
-
Kinetic Energy Of A Spring Equation
May 12, 2025
-
What Are The Predicted Products For The Sn1 Reaction Shown
May 12, 2025
Related Post
Thank you for visiting our website which covers about How Much Atp Is Produced In The Electron Transport Chain . We hope the information provided has been useful to you. Feel free to contact us if you have any questions or need further assistance. See you next time and don't miss to bookmark.